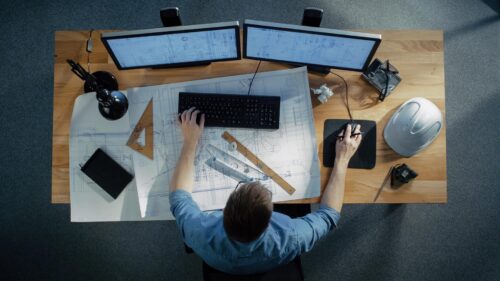
Modeling the DOE’s Energy Infrastructure Reinvestment Program in Resource Planning
Recommendations for utility modelers and regulators to maximize ratepayer savings using low-cost federal loans
Introduction
America’s increasingly electrified economy needs affordable and reliable power in growing amounts. New infrastructure will need to be built to generate and deliver all this electricity, even as legacy grid assets are cost-effectively maintained and, where appropriate, replaced and retired. Even by conservative accounts, the bill for the massive undertaking will be on the order of hundreds of billions of dollars and perhaps reach into the trillions.[1]
Consumers — comprising industry, commerce, and individuals with vastly differing levels of economic security — will ultimately pick up the tab. A critical question is how to protect this diverse group — as a whole and in its constituent parts — from imprudent investment and operational choices that shift inflated costs and unnecessary risks from decision makers to decision takers.
Fortunately, the Inflation Reduction Act of 2022 created a slate of federally funded tools to lower the cost of power sector investments. One of the most important of these tools is the Energy Infrastructure Reinvestment (EIR) program, administered by the Loan Programs Office (LPO) of the US Department of Energy (DOE). The EIR can provide low-interest, guaranteed loans for qualified investments that “retool, repower, repurpose, or replace energy infrastructure that has ceased operations or enable operating energy infrastructure to avoid, reduce, utilize or sequester air pollutants or greenhouse gas emissions.”18 With authority to make up to $250 billion in lending commitments through September 2026 and a $5 billion budget to buy down interest rates below the levels supported by borrowers’ credit ratings alone, the EIR represents an unprecedented (but time-limited) opportunity to secure a least-cost energy future for ratepayers across the country.
There are two key factors that influence how much savings an EIR loan can deliver on a portfolio basis: the first is the composition of investments that are included in a utility’s EIR application, and the second is the financial structure for, and the regulatory treatment of, EIR financing.
Exhibit 1. Factors that Influence EIR Savings Potential: Questions, Solutions, and Guidance
Factor
Question
Solution
Guidance Resource
1) Composition of investments that the utility includes in its EIR application
How might utilities determine the most cost-effective portfolio of resources, considering the unique savings opportunity associated with EIR-eligible resources?
Identify a reinvestment portfolio that maximizes impact of the EIR by incorporating EIR eligibility parameters into capacity expansion modeling.
This brief
2) Financial structure and regulatory treatment of costs associated with an EIR loan
How might utilities structure and recover loan costs to maximize the ratepayer savings from EIR financing?
Apply for a high-leverage EIR loan while insulating the cost of capital for all the utility’s non-EIR assets. Where possible, use EIR loan funds to pay down the balance of risky/retired/retiring utility assets (i.e., capital recycling) to balance ratepayer and shareholder interests.
Maximizing the Value of the Energy Infrastructure Reinvestment Program for Utility Customers
This brief focuses on the first factor, the composition of a truly least-cost resource portfolio. We cover how to integrate the EIR into the parameters for capacity expansion modeling. Certain parameters used for capacity expansion modeling — such as EIR leverage and a utility’s cost of corporate debt and equity in the presence of the EIR — are necessarily impacted by financial structuring and regulatory decisions that we have grouped as the second factor; interested readers should consult RMI’s Maximizing the Value of the Energy Infrastructure Reinvestment Program for Utility Customers for more detail.
Modeling the EIR: A step-by-step approach to a complex task
There are three steps that utility planners and resource modelers can undertake to meaningfully incorporate the EIR into the planning process:
- Model the portfolio-wide savings associated with low-interest-rate financing.
- Model clean repowering — deployment of wind and/or solar plus storage at existing fossil sites, especially sites with low-capacity factor resources such as gas peakers or resources that are set to retire, to more efficiently utilize or retain the existing points of interconnection and accelerate renewables deployment.
- Model potential savings from transmission investment.
These steps reinforce each other by reducing the cost of financing for EIR-eligible assets and shifting the cost-optimal resource portfolio toward EIR-eligible clean resources. Exhibit 2 shows indicative savings realized by modeling each step in capacity expansion. Step 1 applies EIR financing to all clean generation resources. The capacity expansion software chooses a relatively cleaner generation portfolio with greater total build and operating costs. However, increases in build and operating costs are more than offset by financing savings on the clean assets. Step 2 utilizes clean repowering to bypass interconnection constraints and build an even cleaner generation system. The resultant portfolio saves on total build and operating costs. Savings are compounded as an even greater share of generation resources accesses EIR financing. Step 3 applies EIR financing to transmission as well as clean generation. The model selects a resource portfolio with more transmission and clean generation and accordingly less fossil generation. While the total build and operating costs are slightly higher, additional costs are more than offset by savings on clean generation and transmission financing.
How to implement each of these steps in a capacity expansion modeling exercise is discussed in greater detail in the sections that follow, where we also offer recommendations for regulatory action to motivate utilities to undertake these efforts. In a Technical Appendix that follows this brief, we provide additional guidance for planners tasked with developing quantitative inputs for capacity expansion modeling software.
Step 1: Modeling portfolio-wide savings from EIR financing
Regulators across the country are calling on utilities to explore and pursue all possible funding sources that will create benefits for ratepayers. In Washington, for instance, the Utilities and Transportation Commission declared that it expects utilities to evaluate “each IRA and IIJA program to determine their viability and subsequently submit applications for funding where analysis indicates benefit to Washington customers,” specifically recognizing the importance of “an assessment of the EIR loan program as a potential alternative for financing capital investments, including transmission capacity needs.”[3]
To evaluate the EIR opportunity, all utilities should be directed to model the potential portfolio-wide savings from use of the program for all eligible assets, completing the analysis within the next year. This is a minimum step — and one no less prudent than including the impacts of clean energy tax credits in capacity expansion modeling. Just as Production Tax Credit (PTC) and Investment Tax Credit (ITC) parameters will lower the cost of solar, wind, and battery storage and prompt modeling software to select more of those resources, access to preferential financing will improve the cost-effectiveness of eligible assets and favor their deployment, barring other constraints in the model.
To incorporate EIR financing parameters, a modeler can follow these steps:
Step 1.a: Define the financial parameters that are applicable to EIR-eligible resources. The key financial parameters are: 1) a forward EIR interest rate and 2) a ratio of EIR debt to corporate debt and corporate equity. Modelers should estimate a forward EIR interest rate fixed for the life of the loan at the likely future issuance date (before the end of September 2031). The forward interest should represent the federal government’s cost of borrowing for the loan term plus 37.5 basis points. See the Detailed Modeling Instructions appended to this insight brief for more on this topic.
Of course, not all EIR assets will enter service simultaneously or remain in service for the same number of years. For modeling purposes, a single interest rate should be applied to all EIR assets, with a term weighted by initial plant balances (in $) and associated forecasted useful lives, though in no case should the weighted term be longer than the maximum of 30 years allowed under the program. If financing for EIR assets is modeled for a period beyond the EIR loan term, modelers should apply a cost of capital identical to the one applied to non-EIR assets in those same out-years.
- Because debt (both EIR and corporate varieties) has a lower cost of capital than equity, a higher share of debt will tend to yield a lower overall cost of capital (provided there is no offsetting increase in the cost of equity). We encourage consideration of scenarios with higher “leverage” than has traditionally been used in modeling utility capital structures, taking advantage of EIR’s programmatic flexibility to finance up to 80% of project costs with EIR debt. EIR loans can be treated as off-balance sheet obligations to avoid negative repercussions for the utility and ratepayers (i.e., credit downgrades that could increase the cost of non-EIR financing); this topic is explored in detail in RMI’s Maximizing the Value of the Energy Infrastructure Reinvestment Program for Utility Customers.
Step 1.b: Identify the set of resources, technologies, and investments that are eligible for EIR funding and that can be completed by September 30, 2031. Apply the financial parameters determined in step 1.a. to those resource types.
- For initial modeling, all new renewable energy, nuclear, and energy storage technologies can be assumed to qualify for EIR, whether or not they directly replace a retiring fossil asset.[4] We recommended this approach to allow the model to select all cost-effective eligible resources. However, subsequent review of the portfolio will be necessary to confirm the required “nexus” to either reutilizing/repurposing energy infrastructure or enabling existing energy infrastructure to reduce emissions.[5] Discussions with LPO staff can inform the filtering of cost-effective projects that may not be eligible for inclusion in an EIR application.
Step 1.c: Evaluate the impact of incorporating EIR financial parameters into the resource planning model by comparing i) the timing, magnitude, and cost of investments with EIR financing with ii) the timing, magnitude, and cost of investments in a business-as-usual scenario without EIR financing.
Case study: North Carolina Consumer Advocate Modeling of EIR Suggests Baseline Savings of $415 Million
At the time of writing, no utilities have yet incorporated EIR into their IRP modeling. However, the North Carolina Public Staff — the state’s consumer advocate — incorporated portfolio-wide EIR modeling parameters into its modeling in the 2023–2024 Duke Energy Carbon Plan/Integrated Resource Plan. The Public Staff modeled EIR financing of 40% of the total cost of eligible projects placed in service by September 30, 2031. The remaining portion of the capital stack used standard utility financing, proportionally based on the utility’s current corporate debt to equity ratio, leaving the rate of return on corporate debt and equity unchanged. With EIR assumptions added in, the model selected an additional 500 MW of EIR eligible resource capacity through 2032, while simultaneously reducing the present value of the revenue requirement (PVRR) through 2032 by $415 million. Public Staff noted that “EIR also incentivized the model to select solar plus storage over standalone solar, increasing the total capacity of battery storage on the system.”[6]
Recommendations for Regulators:
In cases where the utility has failed to model the EIR program in its resource plan, it is incumbent upon regulators to request — and, preferably, to require — further exploration of this unprecedented opportunity to deliver ratepayer savings. To do so, regulators can request or require that regulated utilities:
- Prepare one or more resource portfolio scenarios that include an EIR interest rate applied portfolio-wide to eligible technologies whose construction can be completed by September 30, 2031. At a minimum, EIR debt can replace corporate debt in the capital stack of the eligible assets. But, to maximize savings, utilities can use EIR debt to replace both corporate debt and corporate equity, with the assumption that the EIR loan will be held off-balance sheet and repaid via dedicated surcharge.[7] Therefore, regulators should request that at least one modeled scenario utilize a ratio of EIR debt that increases the utility’s Commission-approved debt-to-equity ratio, taking advantage of the financial and regulatory structuring flexibility allowed under the EIR. For more detail on this approach, review RMI’s Maximizing the Value of the Energy Infrastructure Reinvestment Program for Utility Customers report.
- Compare the impact of the EIR scenario(s) with all other modeled portfolios along the dimensions of 1) present value of the revenue requirement (PVRR), 2) changes to the timing of investments in different resource types, and 3) annual net capacity changes by resource type.
If a utility has incorporated the EIR into its modeling, it is important to ensure that the program has been simulated accurately. Regulators can ask the utility to explain and justify the assumptions for EIR financial parameters used, and to disclose other relevant parameter assumptions (e.g., regarding asset eligibility and federal compliance costs).
Step 2: Modeling Clean Repowering
Among the wide range of projects eligible for EIR funding, one type merits special consideration: the development of clean energy resources deployed behind existing (often underutilized) points of interconnection for fossil generation, which we refer to as “clean repowering.” Clean repowering investments offer the benefits of faster deployment and economic savings compared to development of projects at new sites. RMI’s analysis suggests EIR-financed clean repowering could support the development of 250 GW of clean energy resources across the United States,[9] yielding annual savings of $12.7 billion for the next decade.[10]
The capacity expansion models utilized by utility planners often ignore clean repowering opportunities, because they consider generic, rather than site-specific, resource additions. However, clean repowering resources provide unique benefits and cost reductions, which may make them cost-effective at higher quantities than generic clean energy additions. To generate lowest-cost resource portfolios, it is advisable for utility planners to specify clean repowering resources in capacity expansion modeling.
Fortunately, utility planners can model clean repowering with minor modifications to existing capacity expansion tools. Solar, wind, and battery storage resources can be added as resource candidates at existing generator sites, subject to the constraint that joint output from the existing and clean repowering resources does not exceed the limits of the point of interconnection. Implementation will vary across modeling software, but typically it will resemble the way many utilities currently model collocated solar and storage. (See the Detailed Modeling Instructions appended to this insight brief for more on this topic.)
To accurately represent clean repowering in capacity expansion software, modelers can take the following steps:
Step 2.a: Remove interconnection delay assumptions from clean repowering resources. Interconnection queue backlogs are lengthening across the nation.[11] To reflect the growing challenges of interconnection, some utility planners are constraining the pace of new clean energy builds in their capacity expansion models. In some cases, the result is that economic clean energy builds are rejected and fossil-fueled generators added in their place. In other cases, clean energy builds are delayed, which might incur project delay costs that increase the total system costs. The costs to ratepayers of such outcomes are even greater when we consider foregone savings from EIR financing for eligible clean assets.[12]
Clean repowering can enable faster interconnection of new clean energy resources by accessing surplus interconnection rules, especially Surplus Interconnection Service as established by Federal Energy Regulatory Commission (FERC) Order 845.
In addition to facilitating more favorable regulatory treatment, clean repowering can also avoid other delays to traditional generator interconnection, since the resources:
- Can be operated within the limits of existing transmission system interconnection, obviating the need for time-consuming infrastructure upgrades;
- Can be added without de-energizing transmission lines, as is sometimes required with establishing new points of interconnection; and
- In some cases, can make use of existing utility-owned assets and be sited on land that is utility owned or controlled, potentially bypassing many project development risks, especially those associated with third-party contracted resources.
It is important that utility planners model the interconnection benefits of clean repowering resources in capacity expansion, either by accelerating build times or raising annual limits on clean resource builds, as appropriate. This will ensure that all feasible cost-effective clean energy builds are included in resource plans (and, therefore, in utility EIR applications to LPO).
Step 2.b: Apply energy community tax credit bonuses for additional cost savings. The Inflation Reduction Act offers additional tax credit bonuses for clean energy resources developed in “energy communities.” Many fossil-fueled generators are located in energy communities. Savings from the energy community tax credit bonus are substantial: up to an additional 10% of project costs if applied to the ITC or an additional 10% of the credit supplied by the PTC. Base tax credits, energy community bonuses, and EIR financing can all stack together, providing savings on top of savings.
Step 2.c: Apply lower transmission interconnection cost assumptions to clean repowering resources. Utilities often model transmission interconnection costs as a component of capital costs in capacity expansion. Because much of the infrastructure required to interconnect clean repowering resources to the transmission system is already in place, costs are likely to be lower for clean repowering resources than greenfield clean energy projects. In its own modeling, RMI assumes that the interconnection costs associated with clean repowering resources are limited to the construction of spur lines to the point of interconnection.[13] Incorporating these savings into the capacity expansion model may result in additional clean energy resources becoming economic, impacting the optimal EIR portfolio and further lowering the present value of the utility’s revenue requirement.
Step 2.d: Set realistic constraints on clean repowering resource availability. Clean repowering is an attractive but limited opportunity. Available wind, solar, and battery storage capacity at each clean repowering site should reflect geographic constraints. RMI’s analysis of the clean repowering opportunity calculated limits using NREL solar and wind supply curves, assuming all clean repowering resources will be located within a 50 km radius of utility-owned existing or retired fossil plants.
Recommendations for Regulators:
Clean repowering represents a novel approach to increasing generation capacity while maximizing existing transmission and distribution infrastructure. Utilities are unlikely to model clean repowering opportunities without either encouragement from regulators or robust leadership on the part of other stakeholders. Under the traditional cost of service utility business model, new transmission lines that allow the utility to interconnect greenfield generation offer the potential for significant additional earnings — provided of course that they can be built — whereas clean repowering offers much less. Clean repowering should be compared with a reasonable counterfactual, often with slower rates of deployment of capital and clean energy due to interconnection and transmission build delays. The accelerated pace of investment possible with the EIR plus clean repowering can make this approach more attractive than alternatives even under the current utility business model.[14]
Regulators can help safeguard ratepayers by requiring a utility to model at least one scenario with clean repowering and the EIR included. Alternatively, prior to an IRP proceeding, regulators might require a utility to analyze its entire service territory and report all cost-effective clean repowering opportunities within their footprint.
Step 3: Model Savings from Transmission Investment
Transmission system investment may be eligible for EIR financing, subject to LPO review of the potential to reduce systemwide greenhouse gas emissions. Eligible investment may include reconductoring of existing lines, deployment of grid-enhancing technologies (GETs), and new transmission infrastructure if it can be linked to enabling the reduction of GHG emissions.
Utilities and their customers generally stand to benefit from including any planned transmission investments in their EIR applications. If the utilities can make the case that these transmission investments facilitate clean energy integration, the cost of developing already-planned transmission investments could be lower.
To maximize customer savings, utilities can consider opportunities to leverage EIR funding to support more ambitious transmission plans that in turn facilitate the integration of additional clean energy generation. Because both the transmission upgrades and clean energy additions are eligible for EIR financing, accelerated deployment plans will be more economically competitive than ever. Depending on data availability, utilities can conduct this analysis in one of the two following ways.
Option 3.a: The first method is to co-optimize transmission investment and generation capacity expansion. This can be done if the utility is able to parametrize the cost of transmission upgrades and implement them directly into generation capacity expansion software. Planners can modify the costs of both transmission investments and clean generation capacity to account for savings from EIR financing, and then let their models determine the optimal portfolio of EIR-financed transmission, EIR-financed clean generation, and utility-financed conventional generation.
Option 3.b: The second method is to test the impacts of utilizing different transmission investment plans as inputs to the generation capacity expansion model. This approach may be more suitable for utilities that conduct transmission and generation capacity expansion planning separately. In this situation, it is worthwhile for planners to test whether access to EIR financing enables a more aggressive transmission investment plan than would otherwise be economic.
Exhibits 3 and 4 below show a stylized example of how EIR financing could alter the optimal transmission investment pathway, in keeping with the second method (Option 3.b) described above. In this example, the utility has modeled two transmission pathways: “Base Transmission (1,000 MW)” and “Aggressive Transmission (1,500 MW).”
Exhibit 3 compares capacity expansion outputs across the two transmission pathways, utilizing utility-only financing.
- Using the Base Transmission case as an input, the capacity expansion model selects 8,000 MW of fossil generation and 2,000 MW of clean generation as the cost-optimal generation portfolio. The total cost of this portfolio is $2,400 million per year, of which $2,200 million is for generation and $200 million for transmission.
- Using the Aggressive Transmission investment plan as an input, the cost-optimal generation portfolio changes: it now comprises 7,000 MW of fossil generation and 4,000 MW of clean generation. Generation costs are $2,050 million per year. Accounting for transmission costs of $400 million per year, the cost of this portfolio is $2,450 million per year.
While the Aggressive Transmission case enables a lower-cost generation capacity expansion plan, total (generation plus transmission) cost is lower under the Base Transmission plan. Therefore, we can expect planners to pick the Base Transmission plan and accompanying fossil-heavy generation mix as the preferred resource portfolio.
Exhibit 4 compares capacity expansion outputs across the two transmission pathways once more, but this time we consider the impacts of including EIR financing on transmission and clean energy expansion. We assume that transmission has an economic lifetime of 40 years, clean resources have an economic lifetime of 20 years, and EIR financing lowers the after-tax financing costs for both types of eligible resources from 10% to 6%. For simplicity, we also assume the total MW buildout of each resource is the same as in the no-EIR financing situation.
Again, we see that the optimal generation portfolio under the Aggressive Transmission case is cleaner and the generation components less costly than under the Base Transmission case. Transmission costs remain larger under the Aggressive Transmission case than under the Base Transmission case. However, with EIR financing, the all-in cost picture has flipped: the Aggressive Transmission pathway, with its greater levels of clean energy integration, is now the lowest-cost option. Taking EIR into account, we can expect planners to advance the Aggressive Transmission case with the cleaner generation mix as their preferred portfolio.
Recommendations for Regulators:
Integrated transmission and generation planning is the gold standard of utility planning.[15] Yet, in many cases, coordination between transmission and generation planning remains limited. Failing to consider how transmission and generation may interact with respect to EIR is likely to lead to an underestimation of EIR-eligible investment, leaving savings on the table. To maximize EIR benefits across transmission and generation, regulators can:
- Request that utilities conduct analysis of planned and potential transmission upgrades (including reconductoring and GETs) as well as additional new lines that have the potential to support greater levels of new resources eligible for the EIR, reporting these findings to the Commission prior to submission of an EIR application; and
- Encourage utilities to model the impacts of the EIR on enhanced transmission investment portfolios, either through co-optimizing transmission investment and generation expansion or through scenario-based analysis.
Time is ticking to apply for EIR
LPO needs at least 6 months and often upward of a year to review applications for funding. Realistically, that means utilities need to apply for EIR funding by the end of 2025 to meet the September 30, 2026, conditional commitment date. But it is critical to recognize that LPO is willing to consider EIR funding for projects which, at the time of application, are at different stages of regulatory consideration and development. To quote from a recent LPO announcement on this topic, applications may:
include a combination of identified near-term projects and less defined, longer-term projects associated with an IRP in a single loan application. Utilities don’t need to wait for regulatory approval to submit an application. Regulatory approvals can occur during the due diligence phase or can be included as conditions precedent or covenants in the loan.2
This LPO guidance underscores the importance of integrated resource planning (IRP) efforts over the next year, in time to inform EIR applications.
The resource planning process is where system needs and economic parameters are optimized. The composition of a least-cost resource portfolio is likely to be significantly affected by the EIR eligibility of the proposed assets as well as the share of EIR debt (i.e., the leverage) assumed for their capital stacks. These factors can be assessed via capacity expansion modeling that is customary in IRP proceedings.
Conclusion
Integrated resource planning is an unparalleled opportunity for utilities, regulators, and stakeholders to prepare the electricity system for future opportunities and risks while also delivering cost-effective service.
RMI has previously called the EIR the “most important clean energy policy you’ve never heard about,” because of its potential to revitalize local communities historically dependent on fossil fuel infrastructure while saving electricity ratepayers money and building new clean energy resources — a triple win for communities, customers, and the climate.[16]
Given the significance of the resource planning process and the time-limited cost-savings potential of the EIR, the two must be mutually informing during the next 12 months. We hope that this insight brief supports utility planners and regulators alike in assessing the maximum savings potential that the EIR can offer their service territories and submitting competitive applications to deliver the savings to ratepayers.
Technical Appendix: Detailed EIR Modeling Instructions for Utility Planners
In the insight brief above, we offer a high-level conceptual approach to incorporating the EIR into a modeling process. This Technical Appendix provides more granular recommendations for how to implement this approach within capacity expansion modeling software.
EIR financing must be reflected in capacity expansion modeling both within the financing parameters and the asset parameters. The purpose of this Technical Appendix is to support utility planners and modelers endeavoring to incorporate the appropriate financing and asset parameters into a modeling software of their choice. Because each modeling software is slightly different, we offer specific yet software-neutral recommendations for implementation. RMI is available for consultation if more explicit modeling guidance is necessary.
Financing parameters
EIR debt is fixed interest rate lending with a maximum term allowed under the law of 30 years, consistent with the forecasted operating life of many utility assets. For some assets (e.g., battery storage), shorter loan terms may be appropriate. Especially long-lived assets (e.g., transmission) should account for a refinancing without EIR financing after 30 years. Thanks to its $5 billion credit subsidy appropriation, DOE is able to offer loans at interest rates as low as 37.5 basis points above the federal government’s cost of borrowing for a similar duration.
Bootstrapping of current Treasury Yield Curves can be used to estimate a forward rate for the appropriate loan term. RMI is available to assist with this calculation, which is laid out below:
- Fit a quadratic equation (ax2 + bx + c) to the Daily Treasury Par Yield Curve Rates, available from the US Department of the Treasury.
- All equations below must be solved iteratively.
- Using the coefficients from the quadratic equation from Step i, calculate:
- Spot rate at issuance = (((Year of Issuance – Year of Yield Curve)2)*a + (Year of Issuance – Year of Yield Curve)*b + c)/100
- Spot rate at Maturity = (((Year of Issuance + Weighted Average Tenor of Loan – Year of Yield Curve)2)*a + (Year of Issuance + Weighted Average Tenor of Loan – Year of Yield Curve)*b + c)/100
- Forward Treasury Rate for Loan = ((1 + Spot Rate at Maturity)^(Year of Issuance + Weighted Average Tenor of Loan – Year of Yield Curve)/(1 + Spot Rate at Issuance )^(Year of Issuance – Year of Yield Curve))^(1/Weighted Average Tenor of Loan) – 1
- Forward EIR Loan Rate = Forward Treasury Rate + 37.5 basis points
- Weighted Average Tenor of Loan = (Face Tenor of Loan +1) – (1+Iterated Value of Forward EIR Loan Rate)/Iterated Value of Forward EIR Loan Rate + Face Tenor of Loan/((1 + Iterated Value of Forward EIR Loan)^(Face Tenor of Loan) – 1)
DOE may make EIR loans for up to 80% of the value of eligible projects, with the balance being provided by the borrower. A combination of 80% EIR lending and 20% utility financing will result in a far lower weighted average cost of capital (WACC) than normal utility financing, which typically combines shareholder equity and corporate debt in roughly equal shares. Critically, EIR projects need to be evaluated for total ratepayer cost using a cost of capital that is distinct from the rate of return applied to non-EIR projects.
RMI recommends that high-leverage EIR loans be structured as off-balance sheet financings without recourse to the utility’s balance sheet in order to insulate the company’s other debt and equity from credit negative cost impacts. Sensitivity testing that accounts for feedback of on-balance sheet EIR lending on the utility’s other financing costs may be appropriate in certain circumstances. RMI is available to consult on this topic.
Modeling guidance:
- Calculate the EIR forward interest rate for the expected loan issuance year for the expected face value of loan term. Weight face value of loan term by Capex of assets to less than 30 years, if suitable.
- Choose an EIR leverage scenario or scenarios.
- For a given leverage scenario, calculate a WACC that includes EIR debt, corporate debt, and corporate equity. To reiterate, the EIR interest rate is fixed for the life of the loan.
- In capacity expansion modeling, apply WACC including EIR debt to eligible assets and WACC without EIR debt to ineligible assets. In some software (such as PLEXOS), this will be done by directly changing the cost of capital input. In others (such as GenX), modelers may need to implement this indirectly, through adjusting the annualized capital costs of candidate resources to account for lower cost of capital.
For EIR transaction costs, there is (per DOE guidance) a facility fee of 0.6% of project costs up to $2 billion, and 0.1% after that initial $2 billion in costs. There are also third-party expenses, which range from $1 to $3 million for projects financed by DOE under Title 17; RMI recommends use of the lower bound of $1 million, as due diligence for EIR projects likely to be far simpler than for non-EIR Title 17 projects, which can have innovative and emerging technologies. These should be treated as up-front costs. There is also an annual EIR maintenance fee ranging from $150,000 to $500,000, depending on the complexity; again, RMI recommends using the lower bound.
Modeling guidance:
- Add up-front transaction costs to EIR asset capital costs, proportional to asset cost.
- Add maintenance fees to operating expenses. In most capacity expansion software, it will be appropriate to input these fees as fixed operations and maintenance (O&M) costs.
Asset parameters
Assets eligible for EIR loans must be tagged to indicate whether the associated financing charges will be calculated with the inclusion of EIR debt or at the unsubsidized utility rate of return. EIR program guidance issued by DOE includes information about eligible project types. In addition, EIR asset costs may need to be adjusted to account for incremental programmatic compliance burdens.
EIR-funded projects must be compliant with the Davis-Bacon Act (i.e., they must pay prevailing wages), National Environmental Policy Act (NEPA), and the Cargo Preference Act. These compliance considerations are discussed at length in Maximizing the Value of the Energy Infrastructure Reinvestment Program for Utility Customers. We offer the following guidance for how to estimate the cost of compliance with these laws:
Davis-Bacon Act compliance costs: In many areas of the country, project cost estimates may already assume the payment of wages similar to those required under Davis-Bacon, especially if unionized labor is the norm. Where this is not the case, since no generally applicable rule can be offered, modelers should seek to establish whether assumed labor costs will differ significantly if EIR financing is employed.
For the weekly payroll requirement, if this capacity is currently lacking, RMI believes it appropriate to assume a single, up-front system upgrade cost that does not scale; once mechanisms are put in place to make one project compliant with weekly payroll processing, the incremental costs for subsequent projects should be negligible.
National Environmental Policy Act (NEPA) review costs: NEPA compliance costs and time delays are likely to be minimal if projects are of types “categorically excluded (CATEX).” In 2024, DOE expanded the categorical exclusions for solar photovoltaic systems on previously disturbed land and for upgrading and rebuilding transmission lines; it also created a categorical exclusion for some energy storage systems (including battery storage) on previously disturbed land.[16] However, some types of projects may trigger more onerous environmental assessments (EA) and Environmental Impact Statements (EIS). EAs typically takes 6 to 9 months, while the even more intensive EIS process, required for projects that will “significantly affect the quality of the human environment,” may take 1.5 to 2 years to complete. Current cost information for performing EA and EIS reviews is hard to come by. A 2014 study by the US Government Accountability Office (GAO) cited DOE data putting the median cost paid to contractors to perform an EIS review from 2003 to 2012 at $1.4 million.[17] Adjusting this figure for inflation from 2007 through 2024 would bring the median cost to $2.1 million. To reiterate, solar, transmission and storage projects on previously disturbed land will very likely qualify for categorical exclusion.[15] In reviewing modeling parameters, regulators should closely scrutinize EA or EIS treatment of asset types covered by an existing DOE CATEX.
Cargo Preference Act compliance costs: Compliance with Cargo Preference necessitates reliance on US-flagged vessels to transport cargo owned, procured, furnished, or financed by the US government. At this point, RMI cannot reasonably gauge the cost impact of the Cargo Preference Act, not least of all because demand for US-flagged shipping will be affected by the number of EIR projects undertaken (by individual utilities and nationwide). Once a baseline for EIR projects in a cost-optimal portfolio for a particular utility is determined, sensitivity analysis should be employed to test the impact of estimated Cargo Preference Act premiums.
Modeling guidance:
- Determine whether asset capex costs must be adjusted to reflect Davis-Bacon wages during construction. An adjustment may also be needed for maintenance capex where those are entered into the model.
- Regarding NEPA, solar and storage projects on previously disturbed land as well as transmission upgrades should, in the opinion of RMI, be treated as categorically excluded, resulting in negligible cost impacts and timing delays. For projects for which a categorical exclusion cannot be plausibly assumed, scenario analyses to account for EA and EIS cost and timing impacts would be reasonable; alternatively, these projects could be excluded from EIR financing treatment.
- For the Cargo Preference Act, RMI recommends modeling the EIR baseline without an explicit cost impact, followed by scenario analysis to test how potential incremental shipping costs might increase capex costs or affect assets and in turn reduce any PVRR advantage of an asset portfolio including EIR financing.
The EIR + Clean Repowering
Clean repowering savings potential will be highly site-specific. Modelers will need to estimate savings from reduced transmission interconnection costs while accounting for the associated operational constraints. A description of the four steps to modeling clean repowering in capacity expansion is available in the main text of this document; more detailed guidance is provided below.
Modeling guidance:
- Create site-specific opportunities for clean repowering resources at existing fossil fuel asset points of interconnection (POI). Wind, solar, and battery storage candidate resources should be made available at the POI, subject to physical and operational constraints.
- Physical constraints: RMI models the potential for unlimited battery storage connections and wind and solar connections capped at the capacities available within a given radius of existing fossil fuel assets, as per NREL’s solar and wind supply curve data. Other assumptions may be made to reflect on-the-ground project development realities known by the utility.
- Operational constraints: The combined output of existing thermal and new clean repowering resources should not exceed the maximum injection limit at the point of interconnection. Implementation of this constraint will vary across capacity expansion software. When possible, it should be done directly, by creating a joint constraint on hourly generation across the resources. It may also be possible to implement indirectly, by creating a new node (or equivalent) at the point of interconnection and instituting a transmission limit to the rest of the network with maximum flow equal to the point of interconnection injection limit. Note that the constraint should be placed on combined resource generation, not installed capacity, as the economically optimal solution to clean repowering may likely be to “overbuild” capacity behind the point of interconnection and use the hybrid resource flexibility to increase resource capacity factor. Enhanced availability from the hybrid resource is also likely to result in greater ability to meet peak demand, which should be reflected in the combined resource’s capacity accreditation.
- Reflect the value of clean repowering resources to mitigate interconnection delays. As described in the body of the main text, clean repowering resources are not subject to many of the interconnection delays faced by greenfield resources. In capacity expansion, the best way to model this is to remove any limits or constraints applied to annual capacity additions for clean repowering resources.
- Capture the economic benefits of clean repowering resources.
- One benefit of clean repowering resources is reduced transmission interconnection costs. In RMI’s modeling, these savings are captured by assuming the only transmission costs associated with the clean repowering resources are the costs of spur lines to connect to the existing thermal generation point of interconnection. Any other balance of system cost savings associated with developing hybrid rather than standalone resources should be reflected as well. All of these savings should be reflected in capacity expansion as reduced capital costs.
- Another economic benefit is the applicability of enhanced tax credits made available by the Inflation Reduction Act. In addition to the base value of the ITC or PTC, many clean repowering resources are likely to be eligible for the Energy Community Tax Credit Bonus given their proximity and intention connection to existing fossil infrastructure. This bonus credit provides up to an additional 10% of project costs if applied to the ITC or an additional 10% of the credit supplied by the PTC. Eligibility maps can be found on the program website. These savings should be modeled in capacity expansion as adjustments to resource capital costs.
- Finally, all clean repowering resources are eligible for EIR financing. These savings should be represented in capacity expansion the same as for other clean assets.
Endnotes
[1] E. Larson, C. Greig, J. Jenkins, E. Mayfield, A. Pascale, C. Zhang, J. Drossman, R. Williams, S. Pacala, R. Socolow, EJ Baik, R. Birdsey, R. Duke, R. Jones, B. Haley, E. Leslie, K. Paustian, and A. Swan, Net-Zero America: Potential Pathways, Infrastructure, and Impacts, Interim Report, Princeton University, 2020, https://netzeroamerica.princeton.edu/the-report.
[2] Support for this assumption is presented in Christian Fong, David Posner, and Uday Varadarajan, “Maximizing the Value of the Energy Infrastructure Reinvestment Program for Utility Customers,” RMI, May 24, 2024, https://rmi.org/maximizing-the-value-of-the-energy-infrastructure-reinvestment-program-for-utility-customers/.
[3] Docket No. E-100, Sub 190, “Testimony of Jeff Thomas Public Staff,” North Carolina Utilities Commission, May 29, 2024, https://starw1.ncuc.gov/NCUC/ViewFile.aspx?Id=eb84ecb4-3891-40a2-a7eb-426b153f1f3a.
[4] According to the US Department of Energy’s Title 17 Loan Program Guidance, to be eligible for EIR funding, investments need only “avoid, reduce, utilize, or sequester air pollutants and anthropogenic emissions of greenhouse gases,” which can include investments in generation resources, as well as a broad array of other technologies such as repowering or retooling energy infrastructure to operate more efficiently or at higher output. However, for the purposes of capacity expansion modeling, we recommend modelers at a minimum assume that all resources that do not emit greenhouse gases be defined as eligible for EIR funding within the model.
[5] Most clean resources selected by a capacity expansion model will likely qualify as “enabling existing energy infrastructure to reduce emissions,” but if these resources differ geographically from the location of existing infrastructure, more effort may be required to ensure compliance with EIR program eligibility requirements. The LPO website stipulates that EIR projects “must show a clear relationship between new services and benefits provided by the Title 17 financed infrastructure and services, and benefits lost from the legacy infrastructure that ceased operations, such as grid capacity, reliability, and workforce retention and opportunities, including if the replacement plan differs from the legacy infrastructure physically and/or geographically.” See https://www.energy.gov/lpo/energy-infrastructure-reinvestment.
[6] Docket U-240013, “Policy Statement Addressing the Federal Inflation Reduction Act and the Infrastructure Investment and Jobs Act in Utility Planning,” Washington Utilities and Transportation Commission, May 3, 2024, https://apiproxy.utc.wa.gov/cases/GetDocument?docID=31&year=2024&docketNumber=240013.
[7] Clean Repowering: How to Capitalize on Fossil Grid Connections to Unlock Clean Energy Growth, RMI, 2024, https://rmi.org/insight/clean-repowering/.
[8] Katie Siegner and Alex Engel, “Clean Repowering: A Near-Term, IRA-Powered Energy Transition Accelerant,” RMI January 16, 2024, https://rmi.org/clean-repowering-a-near-term-ira-powered-energy-transition-accelerant/.
[9] The cost and benefit calculation for project delays is a complex discounting equation. All else equal, delaying deployment should reduce the present value of costs due to discounting. However, there can be cost increases associated with construction financing loan extension, or other payments required for project delays. Additionally, we assume cost of deploying clean energy will decrease as time progresses, but it is uncertain whether the cost savings of postponed deployment will exceed the known savings associated with the EIR program, under which funds are available to be committed between now and September 2026 for projects (or project milestones) that must be completed by September 31, 2031.
[10] “Grid connection backlog grows by 30% in 2023, dominated by requests for solar, wind, and energy storage,” Berkely Lab, April 10, 2024, https://emp.lbl.gov/news/grid-connection-backlog-grows-30-2023-dominated-requests-solar-wind-and-energy-storage.
[11] The Synapse report “No-Regrets Solutions for Accelerating Grid Interconnection How Fast-Track Interconnection Processes Cut Costs in SPP and MISO” provides a real world example of the interconnection cost savings that can be achieved through clean repowering. A solar project in Sumner County, Kansas, had total interconnection costs before construction of approximately $333/kW, of which roughly 70% were for transmission network upgrades, with a wait of more than eight years to interconnect. In contrast, a solar project in Harper County, Kansas, used surplus interconnection, and there were no transmission network upgrades associated with this project, resulting in less than $1/kW total interconnection costs before construction.
[12] RMI’s article “For Ratepayers to Realize Savings from Clean Energy, Utility Business Models Need an Update” elaborates on the ways in which taking advantage of IRA opportunities is misaligned with traditional utility incentives.
[13] As described in documents from the 2021 NARUC-NASEO Comprehensive Electricity Planning Framework Taskforce and 2021 FERC Joint Federal-State Task Force on Electric Transmission. For further discussion and examples of leading practices, see Mark Dyson, Lauren Shwisberg, and Katerina Stephan, Reimagining Resource Planning, RMI, January 2023, https://rmi.org/insight/reimagining-resource-planning/.
[14] Christian Fong, David Posner, and Uday Varadarajan, “The Energy Infrastructure Reinvestment Program: Federal financing for an equitable, clean economy,” RMI, February 16, 2024, https://rmi.org/the-energy-infrastructure-reinvestment-program-federal-financing-for-an-equitable-clean-economy/.
[15] The 2014 GAO report also noted that 95% of DOE’s 9,060 NEPA analyses from 2008 to 2012 were categorical exclusions; ibid., 7.
[16] DOE NEPA Categorical Exclusion Rulemaking, DOE, 2024, https://www.energy.gov/sites/default/files/2024-04/doe-10-cfr-1021-final-rule-2024-04-30-final.pdf.
[17] National Environmental Policy Act: Little Information Exists on NEPA Analyses, GAO-14-369, April 15, 2014, https://www.gao.gov/products/gao-14-370.
[18] LPO’s Energy Infrastructure Reinvestment (1706) Program https://www.energy.gov/lpo/articles/lpos-energy-infrastructure-reinvestment-1706-program
America’s increasingly electrified economy needs affordable and reliable power in growing amounts. New infrastructure will need to be built to generate and deliver all this electricity, even as legacy grid assets are cost-effectively maintained and, where appropriate, replaced and retired. Even by conservative accounts, the bill for the massive undertaking will be on the order of hundreds of billions of dollars and perhaps reach into the trillions.[1]
Consumers — comprising industry, commerce, and individuals with vastly differing levels of economic security — will ultimately pick up the tab. A critical question is how to protect this diverse group — as a whole and in its constituent parts — from imprudent investment and operational choices that shift inflated costs and unnecessary risks from decision makers to decision takers.
Fortunately, the Inflation Reduction Act of 2022 created a slate of federally funded tools to lower the cost of power sector investments. One of the most important of these tools is the Energy Infrastructure Reinvestment (EIR) program, administered by the Loan Programs Office (LPO) of the US Department of Energy (DOE). The EIR can provide low-interest, guaranteed loans for qualified investments that “retool, repower, repurpose, or replace energy infrastructure that has ceased operations or enable operating energy infrastructure to avoid, reduce, utilize or sequester air pollutants or greenhouse gas emissions.”18 With authority to make up to $250 billion in lending commitments through September 2026 and a $5 billion budget to buy down interest rates below the levels supported by borrowers’ credit ratings alone, the EIR represents an unprecedented (but time-limited) opportunity to secure a least-cost energy future for ratepayers across the country.
There are two key factors that influence how much savings an EIR loan can deliver on a portfolio basis: the first is the composition of investments that are included in a utility’s EIR application, and the second is the financial structure for, and the regulatory treatment of, EIR financing.
Exhibit 1. Factors that Influence EIR Savings Potential: Questions, Solutions, and Guidance
Factor | Question | Solution | Guidance Resource |
1) Composition of investments that the utility includes in its EIR application | How might utilities determine the most cost-effective portfolio of resources, considering the unique savings opportunity associated with EIR-eligible resources? | Identify a reinvestment portfolio that maximizes impact of the EIR by incorporating EIR eligibility parameters into capacity expansion modeling. | This brief |
2) Financial structure and regulatory treatment of costs associated with an EIR loan | How might utilities structure and recover loan costs to maximize the ratepayer savings from EIR financing? | Apply for a high-leverage EIR loan while insulating the cost of capital for all the utility’s non-EIR assets. Where possible, use EIR loan funds to pay down the balance of risky/retired/retiring utility assets (i.e., capital recycling) to balance ratepayer and shareholder interests. | Maximizing the Value of the Energy Infrastructure Reinvestment Program for Utility Customers |
This brief focuses on the first factor, the composition of a truly least-cost resource portfolio. We cover how to integrate the EIR into the parameters for capacity expansion modeling. Certain parameters used for capacity expansion modeling — such as EIR leverage and a utility’s cost of corporate debt and equity in the presence of the EIR — are necessarily impacted by financial structuring and regulatory decisions that we have grouped as the second factor; interested readers should consult RMI’s Maximizing the Value of the Energy Infrastructure Reinvestment Program for Utility Customers for more detail.
There are three steps that utility planners and resource modelers can undertake to meaningfully incorporate the EIR into the planning process:
- Model the portfolio-wide savings associated with low-interest-rate financing.
- Model clean repowering — deployment of wind and/or solar plus storage at existing fossil sites, especially sites with low-capacity factor resources such as gas peakers or resources that are set to retire, to more efficiently utilize or retain the existing points of interconnection and accelerate renewables deployment.
- Model potential savings from transmission investment.
These steps reinforce each other by reducing the cost of financing for EIR-eligible assets and shifting the cost-optimal resource portfolio toward EIR-eligible clean resources. Exhibit 2 shows indicative savings realized by modeling each step in capacity expansion. Step 1 applies EIR financing to all clean generation resources. The capacity expansion software chooses a relatively cleaner generation portfolio with greater total build and operating costs. However, increases in build and operating costs are more than offset by financing savings on the clean assets. Step 2 utilizes clean repowering to bypass interconnection constraints and build an even cleaner generation system. The resultant portfolio saves on total build and operating costs. Savings are compounded as an even greater share of generation resources accesses EIR financing. Step 3 applies EIR financing to transmission as well as clean generation. The model selects a resource portfolio with more transmission and clean generation and accordingly less fossil generation. While the total build and operating costs are slightly higher, additional costs are more than offset by savings on clean generation and transmission financing.
How to implement each of these steps in a capacity expansion modeling exercise is discussed in greater detail in the sections that follow, where we also offer recommendations for regulatory action to motivate utilities to undertake these efforts. In a Technical Appendix that follows this brief, we provide additional guidance for planners tasked with developing quantitative inputs for capacity expansion modeling software.
Step 1: Modeling portfolio-wide savings from EIR financing
Regulators across the country are calling on utilities to explore and pursue all possible funding sources that will create benefits for ratepayers. In Washington, for instance, the Utilities and Transportation Commission declared that it expects utilities to evaluate “each IRA and IIJA program to determine their viability and subsequently submit applications for funding where analysis indicates benefit to Washington customers,” specifically recognizing the importance of “an assessment of the EIR loan program as a potential alternative for financing capital investments, including transmission capacity needs.”[3]
To evaluate the EIR opportunity, all utilities should be directed to model the potential portfolio-wide savings from use of the program for all eligible assets, completing the analysis within the next year. This is a minimum step — and one no less prudent than including the impacts of clean energy tax credits in capacity expansion modeling. Just as Production Tax Credit (PTC) and Investment Tax Credit (ITC) parameters will lower the cost of solar, wind, and battery storage and prompt modeling software to select more of those resources, access to preferential financing will improve the cost-effectiveness of eligible assets and favor their deployment, barring other constraints in the model.
To incorporate EIR financing parameters, a modeler can follow these steps:
Step 1.a: Define the financial parameters that are applicable to EIR-eligible resources. The key financial parameters are: 1) a forward EIR interest rate and 2) a ratio of EIR debt to corporate debt and corporate equity. Modelers should estimate a forward EIR interest rate fixed for the life of the loan at the likely future issuance date (before the end of September 2031). The forward interest should represent the federal government’s cost of borrowing for the loan term plus 37.5 basis points. See the Detailed Modeling Instructions appended to this insight brief for more on this topic.
Of course, not all EIR assets will enter service simultaneously or remain in service for the same number of years. For modeling purposes, a single interest rate should be applied to all EIR assets, with a term weighted by initial plant balances (in $) and associated forecasted useful lives, though in no case should the weighted term be longer than the maximum of 30 years allowed under the program. If financing for EIR assets is modeled for a period beyond the EIR loan term, modelers should apply a cost of capital identical to the one applied to non-EIR assets in those same out-years.
- Because debt (both EIR and corporate varieties) has a lower cost of capital than equity, a higher share of debt will tend to yield a lower overall cost of capital (provided there is no offsetting increase in the cost of equity). We encourage consideration of scenarios with higher “leverage” than has traditionally been used in modeling utility capital structures, taking advantage of EIR’s programmatic flexibility to finance up to 80% of project costs with EIR debt. EIR loans can be treated as off-balance sheet obligations to avoid negative repercussions for the utility and ratepayers (i.e., credit downgrades that could increase the cost of non-EIR financing); this topic is explored in detail in RMI’s Maximizing the Value of the Energy Infrastructure Reinvestment Program for Utility Customers.
Step 1.b: Identify the set of resources, technologies, and investments that are eligible for EIR funding and that can be completed by September 30, 2031. Apply the financial parameters determined in step 1.a. to those resource types.
- For initial modeling, all new renewable energy, nuclear, and energy storage technologies can be assumed to qualify for EIR, whether or not they directly replace a retiring fossil asset.[4] We recommended this approach to allow the model to select all cost-effective eligible resources. However, subsequent review of the portfolio will be necessary to confirm the required “nexus” to either reutilizing/repurposing energy infrastructure or enabling existing energy infrastructure to reduce emissions.[5] Discussions with LPO staff can inform the filtering of cost-effective projects that may not be eligible for inclusion in an EIR application.
Step 1.c: Evaluate the impact of incorporating EIR financial parameters into the resource planning model by comparing i) the timing, magnitude, and cost of investments with EIR financing with ii) the timing, magnitude, and cost of investments in a business-as-usual scenario without EIR financing.
Case study: North Carolina Consumer Advocate Modeling of EIR Suggests Baseline Savings of $415 Million
At the time of writing, no utilities have yet incorporated EIR into their IRP modeling. However, the North Carolina Public Staff — the state’s consumer advocate — incorporated portfolio-wide EIR modeling parameters into its modeling in the 2023–2024 Duke Energy Carbon Plan/Integrated Resource Plan. The Public Staff modeled EIR financing of 40% of the total cost of eligible projects placed in service by September 30, 2031. The remaining portion of the capital stack used standard utility financing, proportionally based on the utility’s current corporate debt to equity ratio, leaving the rate of return on corporate debt and equity unchanged. With EIR assumptions added in, the model selected an additional 500 MW of EIR eligible resource capacity through 2032, while simultaneously reducing the present value of the revenue requirement (PVRR) through 2032 by $415 million. Public Staff noted that “EIR also incentivized the model to select solar plus storage over standalone solar, increasing the total capacity of battery storage on the system.”[6]
Recommendations for Regulators:
In cases where the utility has failed to model the EIR program in its resource plan, it is incumbent upon regulators to request — and, preferably, to require — further exploration of this unprecedented opportunity to deliver ratepayer savings. To do so, regulators can request or require that regulated utilities:
- Prepare one or more resource portfolio scenarios that include an EIR interest rate applied portfolio-wide to eligible technologies whose construction can be completed by September 30, 2031. At a minimum, EIR debt can replace corporate debt in the capital stack of the eligible assets. But, to maximize savings, utilities can use EIR debt to replace both corporate debt and corporate equity, with the assumption that the EIR loan will be held off-balance sheet and repaid via dedicated surcharge.[7] Therefore, regulators should request that at least one modeled scenario utilize a ratio of EIR debt that increases the utility’s Commission-approved debt-to-equity ratio, taking advantage of the financial and regulatory structuring flexibility allowed under the EIR. For more detail on this approach, review RMI’s Maximizing the Value of the Energy Infrastructure Reinvestment Program for Utility Customers report.
- Compare the impact of the EIR scenario(s) with all other modeled portfolios along the dimensions of 1) present value of the revenue requirement (PVRR), 2) changes to the timing of investments in different resource types, and 3) annual net capacity changes by resource type.
If a utility has incorporated the EIR into its modeling, it is important to ensure that the program has been simulated accurately. Regulators can ask the utility to explain and justify the assumptions for EIR financial parameters used, and to disclose other relevant parameter assumptions (e.g., regarding asset eligibility and federal compliance costs).
Step 2: Modeling Clean Repowering
Among the wide range of projects eligible for EIR funding, one type merits special consideration: the development of clean energy resources deployed behind existing (often underutilized) points of interconnection for fossil generation, which we refer to as “clean repowering.” Clean repowering investments offer the benefits of faster deployment and economic savings compared to development of projects at new sites. RMI’s analysis suggests EIR-financed clean repowering could support the development of 250 GW of clean energy resources across the United States,[9] yielding annual savings of $12.7 billion for the next decade.[10]
The capacity expansion models utilized by utility planners often ignore clean repowering opportunities, because they consider generic, rather than site-specific, resource additions. However, clean repowering resources provide unique benefits and cost reductions, which may make them cost-effective at higher quantities than generic clean energy additions. To generate lowest-cost resource portfolios, it is advisable for utility planners to specify clean repowering resources in capacity expansion modeling.
Fortunately, utility planners can model clean repowering with minor modifications to existing capacity expansion tools. Solar, wind, and battery storage resources can be added as resource candidates at existing generator sites, subject to the constraint that joint output from the existing and clean repowering resources does not exceed the limits of the point of interconnection. Implementation will vary across modeling software, but typically it will resemble the way many utilities currently model collocated solar and storage. (See the Detailed Modeling Instructions appended to this insight brief for more on this topic.)
To accurately represent clean repowering in capacity expansion software, modelers can take the following steps:
Step 2.a: Remove interconnection delay assumptions from clean repowering resources. Interconnection queue backlogs are lengthening across the nation.[11] To reflect the growing challenges of interconnection, some utility planners are constraining the pace of new clean energy builds in their capacity expansion models. In some cases, the result is that economic clean energy builds are rejected and fossil-fueled generators added in their place. In other cases, clean energy builds are delayed, which might incur project delay costs that increase the total system costs. The costs to ratepayers of such outcomes are even greater when we consider foregone savings from EIR financing for eligible clean assets.[12]
Clean repowering can enable faster interconnection of new clean energy resources by accessing surplus interconnection rules, especially Surplus Interconnection Service as established by Federal Energy Regulatory Commission (FERC) Order 845.
In addition to facilitating more favorable regulatory treatment, clean repowering can also avoid other delays to traditional generator interconnection, since the resources:
- Can be operated within the limits of existing transmission system interconnection, obviating the need for time-consuming infrastructure upgrades;
- Can be added without de-energizing transmission lines, as is sometimes required with establishing new points of interconnection; and
- In some cases, can make use of existing utility-owned assets and be sited on land that is utility owned or controlled, potentially bypassing many project development risks, especially those associated with third-party contracted resources.
It is important that utility planners model the interconnection benefits of clean repowering resources in capacity expansion, either by accelerating build times or raising annual limits on clean resource builds, as appropriate. This will ensure that all feasible cost-effective clean energy builds are included in resource plans (and, therefore, in utility EIR applications to LPO).
Step 2.b: Apply energy community tax credit bonuses for additional cost savings. The Inflation Reduction Act offers additional tax credit bonuses for clean energy resources developed in “energy communities.” Many fossil-fueled generators are located in energy communities. Savings from the energy community tax credit bonus are substantial: up to an additional 10% of project costs if applied to the ITC or an additional 10% of the credit supplied by the PTC. Base tax credits, energy community bonuses, and EIR financing can all stack together, providing savings on top of savings.
Step 2.c: Apply lower transmission interconnection cost assumptions to clean repowering resources. Utilities often model transmission interconnection costs as a component of capital costs in capacity expansion. Because much of the infrastructure required to interconnect clean repowering resources to the transmission system is already in place, costs are likely to be lower for clean repowering resources than greenfield clean energy projects. In its own modeling, RMI assumes that the interconnection costs associated with clean repowering resources are limited to the construction of spur lines to the point of interconnection.[13] Incorporating these savings into the capacity expansion model may result in additional clean energy resources becoming economic, impacting the optimal EIR portfolio and further lowering the present value of the utility’s revenue requirement.
Step 2.d: Set realistic constraints on clean repowering resource availability. Clean repowering is an attractive but limited opportunity. Available wind, solar, and battery storage capacity at each clean repowering site should reflect geographic constraints. RMI’s analysis of the clean repowering opportunity calculated limits using NREL solar and wind supply curves, assuming all clean repowering resources will be located within a 50 km radius of utility-owned existing or retired fossil plants.
Recommendations for Regulators:
Clean repowering represents a novel approach to increasing generation capacity while maximizing existing transmission and distribution infrastructure. Utilities are unlikely to model clean repowering opportunities without either encouragement from regulators or robust leadership on the part of other stakeholders. Under the traditional cost of service utility business model, new transmission lines that allow the utility to interconnect greenfield generation offer the potential for significant additional earnings — provided of course that they can be built — whereas clean repowering offers much less. Clean repowering should be compared with a reasonable counterfactual, often with slower rates of deployment of capital and clean energy due to interconnection and transmission build delays. The accelerated pace of investment possible with the EIR plus clean repowering can make this approach more attractive than alternatives even under the current utility business model.[14]
Regulators can help safeguard ratepayers by requiring a utility to model at least one scenario with clean repowering and the EIR included. Alternatively, prior to an IRP proceeding, regulators might require a utility to analyze its entire service territory and report all cost-effective clean repowering opportunities within their footprint.
Step 3: Model Savings from Transmission Investment
Transmission system investment may be eligible for EIR financing, subject to LPO review of the potential to reduce systemwide greenhouse gas emissions. Eligible investment may include reconductoring of existing lines, deployment of grid-enhancing technologies (GETs), and new transmission infrastructure if it can be linked to enabling the reduction of GHG emissions.
Utilities and their customers generally stand to benefit from including any planned transmission investments in their EIR applications. If the utilities can make the case that these transmission investments facilitate clean energy integration, the cost of developing already-planned transmission investments could be lower.
To maximize customer savings, utilities can consider opportunities to leverage EIR funding to support more ambitious transmission plans that in turn facilitate the integration of additional clean energy generation. Because both the transmission upgrades and clean energy additions are eligible for EIR financing, accelerated deployment plans will be more economically competitive than ever. Depending on data availability, utilities can conduct this analysis in one of the two following ways.
Option 3.a: The first method is to co-optimize transmission investment and generation capacity expansion. This can be done if the utility is able to parametrize the cost of transmission upgrades and implement them directly into generation capacity expansion software. Planners can modify the costs of both transmission investments and clean generation capacity to account for savings from EIR financing, and then let their models determine the optimal portfolio of EIR-financed transmission, EIR-financed clean generation, and utility-financed conventional generation.
Option 3.b: The second method is to test the impacts of utilizing different transmission investment plans as inputs to the generation capacity expansion model. This approach may be more suitable for utilities that conduct transmission and generation capacity expansion planning separately. In this situation, it is worthwhile for planners to test whether access to EIR financing enables a more aggressive transmission investment plan than would otherwise be economic.
Exhibits 3 and 4 below show a stylized example of how EIR financing could alter the optimal transmission investment pathway, in keeping with the second method (Option 3.b) described above. In this example, the utility has modeled two transmission pathways: “Base Transmission (1,000 MW)” and “Aggressive Transmission (1,500 MW).”
Exhibit 3 compares capacity expansion outputs across the two transmission pathways, utilizing utility-only financing.
- Using the Base Transmission case as an input, the capacity expansion model selects 8,000 MW of fossil generation and 2,000 MW of clean generation as the cost-optimal generation portfolio. The total cost of this portfolio is $2,400 million per year, of which $2,200 million is for generation and $200 million for transmission.
- Using the Aggressive Transmission investment plan as an input, the cost-optimal generation portfolio changes: it now comprises 7,000 MW of fossil generation and 4,000 MW of clean generation. Generation costs are $2,050 million per year. Accounting for transmission costs of $400 million per year, the cost of this portfolio is $2,450 million per year.
While the Aggressive Transmission case enables a lower-cost generation capacity expansion plan, total (generation plus transmission) cost is lower under the Base Transmission plan. Therefore, we can expect planners to pick the Base Transmission plan and accompanying fossil-heavy generation mix as the preferred resource portfolio.
Exhibit 4 compares capacity expansion outputs across the two transmission pathways once more, but this time we consider the impacts of including EIR financing on transmission and clean energy expansion. We assume that transmission has an economic lifetime of 40 years, clean resources have an economic lifetime of 20 years, and EIR financing lowers the after-tax financing costs for both types of eligible resources from 10% to 6%. For simplicity, we also assume the total MW buildout of each resource is the same as in the no-EIR financing situation.
Again, we see that the optimal generation portfolio under the Aggressive Transmission case is cleaner and the generation components less costly than under the Base Transmission case. Transmission costs remain larger under the Aggressive Transmission case than under the Base Transmission case. However, with EIR financing, the all-in cost picture has flipped: the Aggressive Transmission pathway, with its greater levels of clean energy integration, is now the lowest-cost option. Taking EIR into account, we can expect planners to advance the Aggressive Transmission case with the cleaner generation mix as their preferred portfolio.
Recommendations for Regulators:
Integrated transmission and generation planning is the gold standard of utility planning.[15] Yet, in many cases, coordination between transmission and generation planning remains limited. Failing to consider how transmission and generation may interact with respect to EIR is likely to lead to an underestimation of EIR-eligible investment, leaving savings on the table. To maximize EIR benefits across transmission and generation, regulators can:
- Request that utilities conduct analysis of planned and potential transmission upgrades (including reconductoring and GETs) as well as additional new lines that have the potential to support greater levels of new resources eligible for the EIR, reporting these findings to the Commission prior to submission of an EIR application; and
- Encourage utilities to model the impacts of the EIR on enhanced transmission investment portfolios, either through co-optimizing transmission investment and generation expansion or through scenario-based analysis.
Time is ticking to apply for EIR
LPO needs at least 6 months and often upward of a year to review applications for funding. Realistically, that means utilities need to apply for EIR funding by the end of 2025 to meet the September 30, 2026, conditional commitment date. But it is critical to recognize that LPO is willing to consider EIR funding for projects which, at the time of application, are at different stages of regulatory consideration and development. To quote from a recent LPO announcement on this topic, applications may:
include a combination of identified near-term projects and less defined, longer-term projects associated with an IRP in a single loan application. Utilities don’t need to wait for regulatory approval to submit an application. Regulatory approvals can occur during the due diligence phase or can be included as conditions precedent or covenants in the loan.2
This LPO guidance underscores the importance of integrated resource planning (IRP) efforts over the next year, in time to inform EIR applications.
The resource planning process is where system needs and economic parameters are optimized. The composition of a least-cost resource portfolio is likely to be significantly affected by the EIR eligibility of the proposed assets as well as the share of EIR debt (i.e., the leverage) assumed for their capital stacks. These factors can be assessed via capacity expansion modeling that is customary in IRP proceedings.
Conclusion
Integrated resource planning is an unparalleled opportunity for utilities, regulators, and stakeholders to prepare the electricity system for future opportunities and risks while also delivering cost-effective service.
RMI has previously called the EIR the “most important clean energy policy you’ve never heard about,” because of its potential to revitalize local communities historically dependent on fossil fuel infrastructure while saving electricity ratepayers money and building new clean energy resources — a triple win for communities, customers, and the climate.[16]
Given the significance of the resource planning process and the time-limited cost-savings potential of the EIR, the two must be mutually informing during the next 12 months. We hope that this insight brief supports utility planners and regulators alike in assessing the maximum savings potential that the EIR can offer their service territories and submitting competitive applications to deliver the savings to ratepayers.
Technical Appendix: Detailed EIR Modeling Instructions for Utility Planners
In the insight brief above, we offer a high-level conceptual approach to incorporating the EIR into a modeling process. This Technical Appendix provides more granular recommendations for how to implement this approach within capacity expansion modeling software.
EIR financing must be reflected in capacity expansion modeling both within the financing parameters and the asset parameters. The purpose of this Technical Appendix is to support utility planners and modelers endeavoring to incorporate the appropriate financing and asset parameters into a modeling software of their choice. Because each modeling software is slightly different, we offer specific yet software-neutral recommendations for implementation. RMI is available for consultation if more explicit modeling guidance is necessary.
Financing parameters
EIR debt is fixed interest rate lending with a maximum term allowed under the law of 30 years, consistent with the forecasted operating life of many utility assets. For some assets (e.g., battery storage), shorter loan terms may be appropriate. Especially long-lived assets (e.g., transmission) should account for a refinancing without EIR financing after 30 years. Thanks to its $5 billion credit subsidy appropriation, DOE is able to offer loans at interest rates as low as 37.5 basis points above the federal government’s cost of borrowing for a similar duration.
Bootstrapping of current Treasury Yield Curves can be used to estimate a forward rate for the appropriate loan term. RMI is available to assist with this calculation, which is laid out below:
- Fit a quadratic equation (ax2 + bx + c) to the Daily Treasury Par Yield Curve Rates, available from the US Department of the Treasury.
- All equations below must be solved iteratively.
- Using the coefficients from the quadratic equation from Step i, calculate:
- Spot rate at issuance = (((Year of Issuance – Year of Yield Curve)2)*a + (Year of Issuance – Year of Yield Curve)*b + c)/100
- Spot rate at Maturity = (((Year of Issuance + Weighted Average Tenor of Loan – Year of Yield Curve)2)*a + (Year of Issuance + Weighted Average Tenor of Loan – Year of Yield Curve)*b + c)/100
- Forward Treasury Rate for Loan = ((1 + Spot Rate at Maturity)^(Year of Issuance + Weighted Average Tenor of Loan – Year of Yield Curve)/(1 + Spot Rate at Issuance )^(Year of Issuance – Year of Yield Curve))^(1/Weighted Average Tenor of Loan) – 1
- Forward EIR Loan Rate = Forward Treasury Rate + 37.5 basis points
- Weighted Average Tenor of Loan = (Face Tenor of Loan +1) – (1+Iterated Value of Forward EIR Loan Rate)/Iterated Value of Forward EIR Loan Rate + Face Tenor of Loan/((1 + Iterated Value of Forward EIR Loan)^(Face Tenor of Loan) – 1)
DOE may make EIR loans for up to 80% of the value of eligible projects, with the balance being provided by the borrower. A combination of 80% EIR lending and 20% utility financing will result in a far lower weighted average cost of capital (WACC) than normal utility financing, which typically combines shareholder equity and corporate debt in roughly equal shares. Critically, EIR projects need to be evaluated for total ratepayer cost using a cost of capital that is distinct from the rate of return applied to non-EIR projects.
RMI recommends that high-leverage EIR loans be structured as off-balance sheet financings without recourse to the utility’s balance sheet in order to insulate the company’s other debt and equity from credit negative cost impacts. Sensitivity testing that accounts for feedback of on-balance sheet EIR lending on the utility’s other financing costs may be appropriate in certain circumstances. RMI is available to consult on this topic.
Modeling guidance:
- Calculate the EIR forward interest rate for the expected loan issuance year for the expected face value of loan term. Weight face value of loan term by Capex of assets to less than 30 years, if suitable.
- Choose an EIR leverage scenario or scenarios.
- For a given leverage scenario, calculate a WACC that includes EIR debt, corporate debt, and corporate equity. To reiterate, the EIR interest rate is fixed for the life of the loan.
- In capacity expansion modeling, apply WACC including EIR debt to eligible assets and WACC without EIR debt to ineligible assets. In some software (such as PLEXOS), this will be done by directly changing the cost of capital input. In others (such as GenX), modelers may need to implement this indirectly, through adjusting the annualized capital costs of candidate resources to account for lower cost of capital.
For EIR transaction costs, there is (per DOE guidance) a facility fee of 0.6% of project costs up to $2 billion, and 0.1% after that initial $2 billion in costs. There are also third-party expenses, which range from $1 to $3 million for projects financed by DOE under Title 17; RMI recommends use of the lower bound of $1 million, as due diligence for EIR projects likely to be far simpler than for non-EIR Title 17 projects, which can have innovative and emerging technologies. These should be treated as up-front costs. There is also an annual EIR maintenance fee ranging from $150,000 to $500,000, depending on the complexity; again, RMI recommends using the lower bound.
Modeling guidance:
- Add up-front transaction costs to EIR asset capital costs, proportional to asset cost.
- Add maintenance fees to operating expenses. In most capacity expansion software, it will be appropriate to input these fees as fixed operations and maintenance (O&M) costs.
Asset parameters
Assets eligible for EIR loans must be tagged to indicate whether the associated financing charges will be calculated with the inclusion of EIR debt or at the unsubsidized utility rate of return. EIR program guidance issued by DOE includes information about eligible project types. In addition, EIR asset costs may need to be adjusted to account for incremental programmatic compliance burdens.
EIR-funded projects must be compliant with the Davis-Bacon Act (i.e., they must pay prevailing wages), National Environmental Policy Act (NEPA), and the Cargo Preference Act. These compliance considerations are discussed at length in Maximizing the Value of the Energy Infrastructure Reinvestment Program for Utility Customers. We offer the following guidance for how to estimate the cost of compliance with these laws:
Davis-Bacon Act compliance costs: In many areas of the country, project cost estimates may already assume the payment of wages similar to those required under Davis-Bacon, especially if unionized labor is the norm. Where this is not the case, since no generally applicable rule can be offered, modelers should seek to establish whether assumed labor costs will differ significantly if EIR financing is employed.
For the weekly payroll requirement, if this capacity is currently lacking, RMI believes it appropriate to assume a single, up-front system upgrade cost that does not scale; once mechanisms are put in place to make one project compliant with weekly payroll processing, the incremental costs for subsequent projects should be negligible.
National Environmental Policy Act (NEPA) review costs: NEPA compliance costs and time delays are likely to be minimal if projects are of types “categorically excluded (CATEX).” In 2024, DOE expanded the categorical exclusions for solar photovoltaic systems on previously disturbed land and for upgrading and rebuilding transmission lines; it also created a categorical exclusion for some energy storage systems (including battery storage) on previously disturbed land.[16] However, some types of projects may trigger more onerous environmental assessments (EA) and Environmental Impact Statements (EIS). EAs typically takes 6 to 9 months, while the even more intensive EIS process, required for projects that will “significantly affect the quality of the human environment,” may take 1.5 to 2 years to complete. Current cost information for performing EA and EIS reviews is hard to come by. A 2014 study by the US Government Accountability Office (GAO) cited DOE data putting the median cost paid to contractors to perform an EIS review from 2003 to 2012 at $1.4 million.[17] Adjusting this figure for inflation from 2007 through 2024 would bring the median cost to $2.1 million. To reiterate, solar, transmission and storage projects on previously disturbed land will very likely qualify for categorical exclusion.[15] In reviewing modeling parameters, regulators should closely scrutinize EA or EIS treatment of asset types covered by an existing DOE CATEX.
Cargo Preference Act compliance costs: Compliance with Cargo Preference necessitates reliance on US-flagged vessels to transport cargo owned, procured, furnished, or financed by the US government. At this point, RMI cannot reasonably gauge the cost impact of the Cargo Preference Act, not least of all because demand for US-flagged shipping will be affected by the number of EIR projects undertaken (by individual utilities and nationwide). Once a baseline for EIR projects in a cost-optimal portfolio for a particular utility is determined, sensitivity analysis should be employed to test the impact of estimated Cargo Preference Act premiums.
Modeling guidance:
- Determine whether asset capex costs must be adjusted to reflect Davis-Bacon wages during construction. An adjustment may also be needed for maintenance capex where those are entered into the model.
- Regarding NEPA, solar and storage projects on previously disturbed land as well as transmission upgrades should, in the opinion of RMI, be treated as categorically excluded, resulting in negligible cost impacts and timing delays. For projects for which a categorical exclusion cannot be plausibly assumed, scenario analyses to account for EA and EIS cost and timing impacts would be reasonable; alternatively, these projects could be excluded from EIR financing treatment.
- For the Cargo Preference Act, RMI recommends modeling the EIR baseline without an explicit cost impact, followed by scenario analysis to test how potential incremental shipping costs might increase capex costs or affect assets and in turn reduce any PVRR advantage of an asset portfolio including EIR financing.
The EIR + Clean Repowering
Clean repowering savings potential will be highly site-specific. Modelers will need to estimate savings from reduced transmission interconnection costs while accounting for the associated operational constraints. A description of the four steps to modeling clean repowering in capacity expansion is available in the main text of this document; more detailed guidance is provided below.
Modeling guidance:
- Create site-specific opportunities for clean repowering resources at existing fossil fuel asset points of interconnection (POI). Wind, solar, and battery storage candidate resources should be made available at the POI, subject to physical and operational constraints.
- Physical constraints: RMI models the potential for unlimited battery storage connections and wind and solar connections capped at the capacities available within a given radius of existing fossil fuel assets, as per NREL’s solar and wind supply curve data. Other assumptions may be made to reflect on-the-ground project development realities known by the utility.
- Operational constraints: The combined output of existing thermal and new clean repowering resources should not exceed the maximum injection limit at the point of interconnection. Implementation of this constraint will vary across capacity expansion software. When possible, it should be done directly, by creating a joint constraint on hourly generation across the resources. It may also be possible to implement indirectly, by creating a new node (or equivalent) at the point of interconnection and instituting a transmission limit to the rest of the network with maximum flow equal to the point of interconnection injection limit. Note that the constraint should be placed on combined resource generation, not installed capacity, as the economically optimal solution to clean repowering may likely be to “overbuild” capacity behind the point of interconnection and use the hybrid resource flexibility to increase resource capacity factor. Enhanced availability from the hybrid resource is also likely to result in greater ability to meet peak demand, which should be reflected in the combined resource’s capacity accreditation.
- Reflect the value of clean repowering resources to mitigate interconnection delays. As described in the body of the main text, clean repowering resources are not subject to many of the interconnection delays faced by greenfield resources. In capacity expansion, the best way to model this is to remove any limits or constraints applied to annual capacity additions for clean repowering resources.
- Capture the economic benefits of clean repowering resources.
- One benefit of clean repowering resources is reduced transmission interconnection costs. In RMI’s modeling, these savings are captured by assuming the only transmission costs associated with the clean repowering resources are the costs of spur lines to connect to the existing thermal generation point of interconnection. Any other balance of system cost savings associated with developing hybrid rather than standalone resources should be reflected as well. All of these savings should be reflected in capacity expansion as reduced capital costs.
- Another economic benefit is the applicability of enhanced tax credits made available by the Inflation Reduction Act. In addition to the base value of the ITC or PTC, many clean repowering resources are likely to be eligible for the Energy Community Tax Credit Bonus given their proximity and intention connection to existing fossil infrastructure. This bonus credit provides up to an additional 10% of project costs if applied to the ITC or an additional 10% of the credit supplied by the PTC. Eligibility maps can be found on the program website. These savings should be modeled in capacity expansion as adjustments to resource capital costs.
- Finally, all clean repowering resources are eligible for EIR financing. These savings should be represented in capacity expansion the same as for other clean assets.
Endnotes
[1] E. Larson, C. Greig, J. Jenkins, E. Mayfield, A. Pascale, C. Zhang, J. Drossman, R. Williams, S. Pacala, R. Socolow, EJ Baik, R. Birdsey, R. Duke, R. Jones, B. Haley, E. Leslie, K. Paustian, and A. Swan, Net-Zero America: Potential Pathways, Infrastructure, and Impacts, Interim Report, Princeton University, 2020, https://netzeroamerica.princeton.edu/the-report.
[2] Support for this assumption is presented in Christian Fong, David Posner, and Uday Varadarajan, “Maximizing the Value of the Energy Infrastructure Reinvestment Program for Utility Customers,” RMI, May 24, 2024, https://rmi.org/maximizing-the-value-of-the-energy-infrastructure-reinvestment-program-for-utility-customers/.
[3] Docket No. E-100, Sub 190, “Testimony of Jeff Thomas Public Staff,” North Carolina Utilities Commission, May 29, 2024, https://starw1.ncuc.gov/NCUC/ViewFile.aspx?Id=eb84ecb4-3891-40a2-a7eb-426b153f1f3a.
[4] According to the US Department of Energy’s Title 17 Loan Program Guidance, to be eligible for EIR funding, investments need only “avoid, reduce, utilize, or sequester air pollutants and anthropogenic emissions of greenhouse gases,” which can include investments in generation resources, as well as a broad array of other technologies such as repowering or retooling energy infrastructure to operate more efficiently or at higher output. However, for the purposes of capacity expansion modeling, we recommend modelers at a minimum assume that all resources that do not emit greenhouse gases be defined as eligible for EIR funding within the model.
[5] Most clean resources selected by a capacity expansion model will likely qualify as “enabling existing energy infrastructure to reduce emissions,” but if these resources differ geographically from the location of existing infrastructure, more effort may be required to ensure compliance with EIR program eligibility requirements. The LPO website stipulates that EIR projects “must show a clear relationship between new services and benefits provided by the Title 17 financed infrastructure and services, and benefits lost from the legacy infrastructure that ceased operations, such as grid capacity, reliability, and workforce retention and opportunities, including if the replacement plan differs from the legacy infrastructure physically and/or geographically.” See https://www.energy.gov/lpo/energy-infrastructure-reinvestment.
[6] Docket U-240013, “Policy Statement Addressing the Federal Inflation Reduction Act and the Infrastructure Investment and Jobs Act in Utility Planning,” Washington Utilities and Transportation Commission, May 3, 2024, https://apiproxy.utc.wa.gov/cases/GetDocument?docID=31&year=2024&docketNumber=240013.
[7] Clean Repowering: How to Capitalize on Fossil Grid Connections to Unlock Clean Energy Growth, RMI, 2024, https://rmi.org/insight/clean-repowering/.
[8] Katie Siegner and Alex Engel, “Clean Repowering: A Near-Term, IRA-Powered Energy Transition Accelerant,” RMI January 16, 2024, https://rmi.org/clean-repowering-a-near-term-ira-powered-energy-transition-accelerant/.
[9] The cost and benefit calculation for project delays is a complex discounting equation. All else equal, delaying deployment should reduce the present value of costs due to discounting. However, there can be cost increases associated with construction financing loan extension, or other payments required for project delays. Additionally, we assume cost of deploying clean energy will decrease as time progresses, but it is uncertain whether the cost savings of postponed deployment will exceed the known savings associated with the EIR program, under which funds are available to be committed between now and September 2026 for projects (or project milestones) that must be completed by September 31, 2031.
[10] “Grid connection backlog grows by 30% in 2023, dominated by requests for solar, wind, and energy storage,” Berkely Lab, April 10, 2024, https://emp.lbl.gov/news/grid-connection-backlog-grows-30-2023-dominated-requests-solar-wind-and-energy-storage.
[11] The Synapse report “No-Regrets Solutions for Accelerating Grid Interconnection How Fast-Track Interconnection Processes Cut Costs in SPP and MISO” provides a real world example of the interconnection cost savings that can be achieved through clean repowering. A solar project in Sumner County, Kansas, had total interconnection costs before construction of approximately $333/kW, of which roughly 70% were for transmission network upgrades, with a wait of more than eight years to interconnect. In contrast, a solar project in Harper County, Kansas, used surplus interconnection, and there were no transmission network upgrades associated with this project, resulting in less than $1/kW total interconnection costs before construction.
[12] RMI’s article “For Ratepayers to Realize Savings from Clean Energy, Utility Business Models Need an Update” elaborates on the ways in which taking advantage of IRA opportunities is misaligned with traditional utility incentives.
[13] As described in documents from the 2021 NARUC-NASEO Comprehensive Electricity Planning Framework Taskforce and 2021 FERC Joint Federal-State Task Force on Electric Transmission. For further discussion and examples of leading practices, see Mark Dyson, Lauren Shwisberg, and Katerina Stephan, Reimagining Resource Planning, RMI, January 2023, https://rmi.org/insight/reimagining-resource-planning/.
[14] Christian Fong, David Posner, and Uday Varadarajan, “The Energy Infrastructure Reinvestment Program: Federal financing for an equitable, clean economy,” RMI, February 16, 2024, https://rmi.org/the-energy-infrastructure-reinvestment-program-federal-financing-for-an-equitable-clean-economy/.
[15] The 2014 GAO report also noted that 95% of DOE’s 9,060 NEPA analyses from 2008 to 2012 were categorical exclusions; ibid., 7.
[16] DOE NEPA Categorical Exclusion Rulemaking, DOE, 2024, https://www.energy.gov/sites/default/files/2024-04/doe-10-cfr-1021-final-rule-2024-04-30-final.pdf.
[17] National Environmental Policy Act: Little Information Exists on NEPA Analyses, GAO-14-369, April 15, 2014, https://www.gao.gov/products/gao-14-370.
[18] LPO’s Energy Infrastructure Reinvestment (1706) Program https://www.energy.gov/lpo/articles/lpos-energy-infrastructure-reinvestment-1706-program
Regulators across the country are calling on utilities to explore and pursue all possible funding sources that will create benefits for ratepayers. In Washington, for instance, the Utilities and Transportation Commission declared that it expects utilities to evaluate “each IRA and IIJA program to determine their viability and subsequently submit applications for funding where analysis indicates benefit to Washington customers,” specifically recognizing the importance of “an assessment of the EIR loan program as a potential alternative for financing capital investments, including transmission capacity needs.”[3]
To evaluate the EIR opportunity, all utilities should be directed to model the potential portfolio-wide savings from use of the program for all eligible assets, completing the analysis within the next year. This is a minimum step — and one no less prudent than including the impacts of clean energy tax credits in capacity expansion modeling. Just as Production Tax Credit (PTC) and Investment Tax Credit (ITC) parameters will lower the cost of solar, wind, and battery storage and prompt modeling software to select more of those resources, access to preferential financing will improve the cost-effectiveness of eligible assets and favor their deployment, barring other constraints in the model.
To incorporate EIR financing parameters, a modeler can follow these steps:
Step 1.a: Define the financial parameters that are applicable to EIR-eligible resources. The key financial parameters are: 1) a forward EIR interest rate and 2) a ratio of EIR debt to corporate debt and corporate equity. Modelers should estimate a forward EIR interest rate fixed for the life of the loan at the likely future issuance date (before the end of September 2031). The forward interest should represent the federal government’s cost of borrowing for the loan term plus 37.5 basis points. See the Detailed Modeling Instructions appended to this insight brief for more on this topic.
Of course, not all EIR assets will enter service simultaneously or remain in service for the same number of years. For modeling purposes, a single interest rate should be applied to all EIR assets, with a term weighted by initial plant balances (in $) and associated forecasted useful lives, though in no case should the weighted term be longer than the maximum of 30 years allowed under the program. If financing for EIR assets is modeled for a period beyond the EIR loan term, modelers should apply a cost of capital identical to the one applied to non-EIR assets in those same out-years.
- Because debt (both EIR and corporate varieties) has a lower cost of capital than equity, a higher share of debt will tend to yield a lower overall cost of capital (provided there is no offsetting increase in the cost of equity). We encourage consideration of scenarios with higher “leverage” than has traditionally been used in modeling utility capital structures, taking advantage of EIR’s programmatic flexibility to finance up to 80% of project costs with EIR debt. EIR loans can be treated as off-balance sheet obligations to avoid negative repercussions for the utility and ratepayers (i.e., credit downgrades that could increase the cost of non-EIR financing); this topic is explored in detail in RMI’s Maximizing the Value of the Energy Infrastructure Reinvestment Program for Utility Customers.
Step 1.b: Identify the set of resources, technologies, and investments that are eligible for EIR funding and that can be completed by September 30, 2031. Apply the financial parameters determined in step 1.a. to those resource types.
- For initial modeling, all new renewable energy, nuclear, and energy storage technologies can be assumed to qualify for EIR, whether or not they directly replace a retiring fossil asset.[4] We recommended this approach to allow the model to select all cost-effective eligible resources. However, subsequent review of the portfolio will be necessary to confirm the required “nexus” to either reutilizing/repurposing energy infrastructure or enabling existing energy infrastructure to reduce emissions.[5] Discussions with LPO staff can inform the filtering of cost-effective projects that may not be eligible for inclusion in an EIR application.
Step 1.c: Evaluate the impact of incorporating EIR financial parameters into the resource planning model by comparing i) the timing, magnitude, and cost of investments with EIR financing with ii) the timing, magnitude, and cost of investments in a business-as-usual scenario without EIR financing.
At the time of writing, no utilities have yet incorporated EIR into their IRP modeling. However, the North Carolina Public Staff — the state’s consumer advocate — incorporated portfolio-wide EIR modeling parameters into its modeling in the 2023–2024 Duke Energy Carbon Plan/Integrated Resource Plan. The Public Staff modeled EIR financing of 40% of the total cost of eligible projects placed in service by September 30, 2031. The remaining portion of the capital stack used standard utility financing, proportionally based on the utility’s current corporate debt to equity ratio, leaving the rate of return on corporate debt and equity unchanged. With EIR assumptions added in, the model selected an additional 500 MW of EIR eligible resource capacity through 2032, while simultaneously reducing the present value of the revenue requirement (PVRR) through 2032 by $415 million. Public Staff noted that “EIR also incentivized the model to select solar plus storage over standalone solar, increasing the total capacity of battery storage on the system.”[6]
Recommendations for Regulators:
In cases where the utility has failed to model the EIR program in its resource plan, it is incumbent upon regulators to request — and, preferably, to require — further exploration of this unprecedented opportunity to deliver ratepayer savings. To do so, regulators can request or require that regulated utilities:
- Prepare one or more resource portfolio scenarios that include an EIR interest rate applied portfolio-wide to eligible technologies whose construction can be completed by September 30, 2031. At a minimum, EIR debt can replace corporate debt in the capital stack of the eligible assets. But, to maximize savings, utilities can use EIR debt to replace both corporate debt and corporate equity, with the assumption that the EIR loan will be held off-balance sheet and repaid via dedicated surcharge.[7] Therefore, regulators should request that at least one modeled scenario utilize a ratio of EIR debt that increases the utility’s Commission-approved debt-to-equity ratio, taking advantage of the financial and regulatory structuring flexibility allowed under the EIR. For more detail on this approach, review RMI’s Maximizing the Value of the Energy Infrastructure Reinvestment Program for Utility Customers report.
- Compare the impact of the EIR scenario(s) with all other modeled portfolios along the dimensions of 1) present value of the revenue requirement (PVRR), 2) changes to the timing of investments in different resource types, and 3) annual net capacity changes by resource type.
If a utility has incorporated the EIR into its modeling, it is important to ensure that the program has been simulated accurately. Regulators can ask the utility to explain and justify the assumptions for EIR financial parameters used, and to disclose other relevant parameter assumptions (e.g., regarding asset eligibility and federal compliance costs).
Among the wide range of projects eligible for EIR funding, one type merits special consideration: the development of clean energy resources deployed behind existing (often underutilized) points of interconnection for fossil generation, which we refer to as “clean repowering.” Clean repowering investments offer the benefits of faster deployment and economic savings compared to development of projects at new sites. RMI’s analysis suggests EIR-financed clean repowering could support the development of 250 GW of clean energy resources across the United States,[9] yielding annual savings of $12.7 billion for the next decade.[10]
The capacity expansion models utilized by utility planners often ignore clean repowering opportunities, because they consider generic, rather than site-specific, resource additions. However, clean repowering resources provide unique benefits and cost reductions, which may make them cost-effective at higher quantities than generic clean energy additions. To generate lowest-cost resource portfolios, it is advisable for utility planners to specify clean repowering resources in capacity expansion modeling.
Fortunately, utility planners can model clean repowering with minor modifications to existing capacity expansion tools. Solar, wind, and battery storage resources can be added as resource candidates at existing generator sites, subject to the constraint that joint output from the existing and clean repowering resources does not exceed the limits of the point of interconnection. Implementation will vary across modeling software, but typically it will resemble the way many utilities currently model collocated solar and storage. (See the Detailed Modeling Instructions appended to this insight brief for more on this topic.)
To accurately represent clean repowering in capacity expansion software, modelers can take the following steps:
Step 2.a: Remove interconnection delay assumptions from clean repowering resources. Interconnection queue backlogs are lengthening across the nation.[11] To reflect the growing challenges of interconnection, some utility planners are constraining the pace of new clean energy builds in their capacity expansion models. In some cases, the result is that economic clean energy builds are rejected and fossil-fueled generators added in their place. In other cases, clean energy builds are delayed, which might incur project delay costs that increase the total system costs. The costs to ratepayers of such outcomes are even greater when we consider foregone savings from EIR financing for eligible clean assets.[12]
Clean repowering can enable faster interconnection of new clean energy resources by accessing surplus interconnection rules, especially Surplus Interconnection Service as established by Federal Energy Regulatory Commission (FERC) Order 845.
In addition to facilitating more favorable regulatory treatment, clean repowering can also avoid other delays to traditional generator interconnection, since the resources:
- Can be operated within the limits of existing transmission system interconnection, obviating the need for time-consuming infrastructure upgrades;
- Can be added without de-energizing transmission lines, as is sometimes required with establishing new points of interconnection; and
- In some cases, can make use of existing utility-owned assets and be sited on land that is utility owned or controlled, potentially bypassing many project development risks, especially those associated with third-party contracted resources.
It is important that utility planners model the interconnection benefits of clean repowering resources in capacity expansion, either by accelerating build times or raising annual limits on clean resource builds, as appropriate. This will ensure that all feasible cost-effective clean energy builds are included in resource plans (and, therefore, in utility EIR applications to LPO).
Step 2.b: Apply energy community tax credit bonuses for additional cost savings. The Inflation Reduction Act offers additional tax credit bonuses for clean energy resources developed in “energy communities.” Many fossil-fueled generators are located in energy communities. Savings from the energy community tax credit bonus are substantial: up to an additional 10% of project costs if applied to the ITC or an additional 10% of the credit supplied by the PTC. Base tax credits, energy community bonuses, and EIR financing can all stack together, providing savings on top of savings.
Step 2.c: Apply lower transmission interconnection cost assumptions to clean repowering resources. Utilities often model transmission interconnection costs as a component of capital costs in capacity expansion. Because much of the infrastructure required to interconnect clean repowering resources to the transmission system is already in place, costs are likely to be lower for clean repowering resources than greenfield clean energy projects. In its own modeling, RMI assumes that the interconnection costs associated with clean repowering resources are limited to the construction of spur lines to the point of interconnection.[13] Incorporating these savings into the capacity expansion model may result in additional clean energy resources becoming economic, impacting the optimal EIR portfolio and further lowering the present value of the utility’s revenue requirement.
Step 2.d: Set realistic constraints on clean repowering resource availability. Clean repowering is an attractive but limited opportunity. Available wind, solar, and battery storage capacity at each clean repowering site should reflect geographic constraints. RMI’s analysis of the clean repowering opportunity calculated limits using NREL solar and wind supply curves, assuming all clean repowering resources will be located within a 50 km radius of utility-owned existing or retired fossil plants.
Recommendations for Regulators:
Clean repowering represents a novel approach to increasing generation capacity while maximizing existing transmission and distribution infrastructure. Utilities are unlikely to model clean repowering opportunities without either encouragement from regulators or robust leadership on the part of other stakeholders. Under the traditional cost of service utility business model, new transmission lines that allow the utility to interconnect greenfield generation offer the potential for significant additional earnings — provided of course that they can be built — whereas clean repowering offers much less. Clean repowering should be compared with a reasonable counterfactual, often with slower rates of deployment of capital and clean energy due to interconnection and transmission build delays. The accelerated pace of investment possible with the EIR plus clean repowering can make this approach more attractive than alternatives even under the current utility business model.[14]
Regulators can help safeguard ratepayers by requiring a utility to model at least one scenario with clean repowering and the EIR included. Alternatively, prior to an IRP proceeding, regulators might require a utility to analyze its entire service territory and report all cost-effective clean repowering opportunities within their footprint.
Step 3: Model Savings from Transmission Investment
Transmission system investment may be eligible for EIR financing, subject to LPO review of the potential to reduce systemwide greenhouse gas emissions. Eligible investment may include reconductoring of existing lines, deployment of grid-enhancing technologies (GETs), and new transmission infrastructure if it can be linked to enabling the reduction of GHG emissions.
Utilities and their customers generally stand to benefit from including any planned transmission investments in their EIR applications. If the utilities can make the case that these transmission investments facilitate clean energy integration, the cost of developing already-planned transmission investments could be lower.
To maximize customer savings, utilities can consider opportunities to leverage EIR funding to support more ambitious transmission plans that in turn facilitate the integration of additional clean energy generation. Because both the transmission upgrades and clean energy additions are eligible for EIR financing, accelerated deployment plans will be more economically competitive than ever. Depending on data availability, utilities can conduct this analysis in one of the two following ways.
Option 3.a: The first method is to co-optimize transmission investment and generation capacity expansion. This can be done if the utility is able to parametrize the cost of transmission upgrades and implement them directly into generation capacity expansion software. Planners can modify the costs of both transmission investments and clean generation capacity to account for savings from EIR financing, and then let their models determine the optimal portfolio of EIR-financed transmission, EIR-financed clean generation, and utility-financed conventional generation.
Option 3.b: The second method is to test the impacts of utilizing different transmission investment plans as inputs to the generation capacity expansion model. This approach may be more suitable for utilities that conduct transmission and generation capacity expansion planning separately. In this situation, it is worthwhile for planners to test whether access to EIR financing enables a more aggressive transmission investment plan than would otherwise be economic.
Exhibits 3 and 4 below show a stylized example of how EIR financing could alter the optimal transmission investment pathway, in keeping with the second method (Option 3.b) described above. In this example, the utility has modeled two transmission pathways: “Base Transmission (1,000 MW)” and “Aggressive Transmission (1,500 MW).”
Exhibit 3 compares capacity expansion outputs across the two transmission pathways, utilizing utility-only financing.
- Using the Base Transmission case as an input, the capacity expansion model selects 8,000 MW of fossil generation and 2,000 MW of clean generation as the cost-optimal generation portfolio. The total cost of this portfolio is $2,400 million per year, of which $2,200 million is for generation and $200 million for transmission.
- Using the Aggressive Transmission investment plan as an input, the cost-optimal generation portfolio changes: it now comprises 7,000 MW of fossil generation and 4,000 MW of clean generation. Generation costs are $2,050 million per year. Accounting for transmission costs of $400 million per year, the cost of this portfolio is $2,450 million per year.
While the Aggressive Transmission case enables a lower-cost generation capacity expansion plan, total (generation plus transmission) cost is lower under the Base Transmission plan. Therefore, we can expect planners to pick the Base Transmission plan and accompanying fossil-heavy generation mix as the preferred resource portfolio.
Exhibit 4 compares capacity expansion outputs across the two transmission pathways once more, but this time we consider the impacts of including EIR financing on transmission and clean energy expansion. We assume that transmission has an economic lifetime of 40 years, clean resources have an economic lifetime of 20 years, and EIR financing lowers the after-tax financing costs for both types of eligible resources from 10% to 6%. For simplicity, we also assume the total MW buildout of each resource is the same as in the no-EIR financing situation.
Again, we see that the optimal generation portfolio under the Aggressive Transmission case is cleaner and the generation components less costly than under the Base Transmission case. Transmission costs remain larger under the Aggressive Transmission case than under the Base Transmission case. However, with EIR financing, the all-in cost picture has flipped: the Aggressive Transmission pathway, with its greater levels of clean energy integration, is now the lowest-cost option. Taking EIR into account, we can expect planners to advance the Aggressive Transmission case with the cleaner generation mix as their preferred portfolio.
Recommendations for Regulators:
Integrated transmission and generation planning is the gold standard of utility planning.[15] Yet, in many cases, coordination between transmission and generation planning remains limited. Failing to consider how transmission and generation may interact with respect to EIR is likely to lead to an underestimation of EIR-eligible investment, leaving savings on the table. To maximize EIR benefits across transmission and generation, regulators can:
- Request that utilities conduct analysis of planned and potential transmission upgrades (including reconductoring and GETs) as well as additional new lines that have the potential to support greater levels of new resources eligible for the EIR, reporting these findings to the Commission prior to submission of an EIR application; and
- Encourage utilities to model the impacts of the EIR on enhanced transmission investment portfolios, either through co-optimizing transmission investment and generation expansion or through scenario-based analysis.
Time is ticking to apply for EIR
LPO needs at least 6 months and often upward of a year to review applications for funding. Realistically, that means utilities need to apply for EIR funding by the end of 2025 to meet the September 30, 2026, conditional commitment date. But it is critical to recognize that LPO is willing to consider EIR funding for projects which, at the time of application, are at different stages of regulatory consideration and development. To quote from a recent LPO announcement on this topic, applications may:
include a combination of identified near-term projects and less defined, longer-term projects associated with an IRP in a single loan application. Utilities don’t need to wait for regulatory approval to submit an application. Regulatory approvals can occur during the due diligence phase or can be included as conditions precedent or covenants in the loan.2
This LPO guidance underscores the importance of integrated resource planning (IRP) efforts over the next year, in time to inform EIR applications.
The resource planning process is where system needs and economic parameters are optimized. The composition of a least-cost resource portfolio is likely to be significantly affected by the EIR eligibility of the proposed assets as well as the share of EIR debt (i.e., the leverage) assumed for their capital stacks. These factors can be assessed via capacity expansion modeling that is customary in IRP proceedings.
Conclusion
Integrated resource planning is an unparalleled opportunity for utilities, regulators, and stakeholders to prepare the electricity system for future opportunities and risks while also delivering cost-effective service.
RMI has previously called the EIR the “most important clean energy policy you’ve never heard about,” because of its potential to revitalize local communities historically dependent on fossil fuel infrastructure while saving electricity ratepayers money and building new clean energy resources — a triple win for communities, customers, and the climate.[16]
Given the significance of the resource planning process and the time-limited cost-savings potential of the EIR, the two must be mutually informing during the next 12 months. We hope that this insight brief supports utility planners and regulators alike in assessing the maximum savings potential that the EIR can offer their service territories and submitting competitive applications to deliver the savings to ratepayers.
Technical Appendix: Detailed EIR Modeling Instructions for Utility Planners
In the insight brief above, we offer a high-level conceptual approach to incorporating the EIR into a modeling process. This Technical Appendix provides more granular recommendations for how to implement this approach within capacity expansion modeling software.
EIR financing must be reflected in capacity expansion modeling both within the financing parameters and the asset parameters. The purpose of this Technical Appendix is to support utility planners and modelers endeavoring to incorporate the appropriate financing and asset parameters into a modeling software of their choice. Because each modeling software is slightly different, we offer specific yet software-neutral recommendations for implementation. RMI is available for consultation if more explicit modeling guidance is necessary.
Financing parameters
EIR debt is fixed interest rate lending with a maximum term allowed under the law of 30 years, consistent with the forecasted operating life of many utility assets. For some assets (e.g., battery storage), shorter loan terms may be appropriate. Especially long-lived assets (e.g., transmission) should account for a refinancing without EIR financing after 30 years. Thanks to its $5 billion credit subsidy appropriation, DOE is able to offer loans at interest rates as low as 37.5 basis points above the federal government’s cost of borrowing for a similar duration.
Bootstrapping of current Treasury Yield Curves can be used to estimate a forward rate for the appropriate loan term. RMI is available to assist with this calculation, which is laid out below:
- Fit a quadratic equation (ax2 + bx + c) to the Daily Treasury Par Yield Curve Rates, available from the US Department of the Treasury.
- All equations below must be solved iteratively.
- Using the coefficients from the quadratic equation from Step i, calculate:
- Spot rate at issuance = (((Year of Issuance – Year of Yield Curve)2)*a + (Year of Issuance – Year of Yield Curve)*b + c)/100
- Spot rate at Maturity = (((Year of Issuance + Weighted Average Tenor of Loan – Year of Yield Curve)2)*a + (Year of Issuance + Weighted Average Tenor of Loan – Year of Yield Curve)*b + c)/100
- Forward Treasury Rate for Loan = ((1 + Spot Rate at Maturity)^(Year of Issuance + Weighted Average Tenor of Loan – Year of Yield Curve)/(1 + Spot Rate at Issuance )^(Year of Issuance – Year of Yield Curve))^(1/Weighted Average Tenor of Loan) – 1
- Forward EIR Loan Rate = Forward Treasury Rate + 37.5 basis points
- Weighted Average Tenor of Loan = (Face Tenor of Loan +1) – (1+Iterated Value of Forward EIR Loan Rate)/Iterated Value of Forward EIR Loan Rate + Face Tenor of Loan/((1 + Iterated Value of Forward EIR Loan)^(Face Tenor of Loan) – 1)
DOE may make EIR loans for up to 80% of the value of eligible projects, with the balance being provided by the borrower. A combination of 80% EIR lending and 20% utility financing will result in a far lower weighted average cost of capital (WACC) than normal utility financing, which typically combines shareholder equity and corporate debt in roughly equal shares. Critically, EIR projects need to be evaluated for total ratepayer cost using a cost of capital that is distinct from the rate of return applied to non-EIR projects.
RMI recommends that high-leverage EIR loans be structured as off-balance sheet financings without recourse to the utility’s balance sheet in order to insulate the company’s other debt and equity from credit negative cost impacts. Sensitivity testing that accounts for feedback of on-balance sheet EIR lending on the utility’s other financing costs may be appropriate in certain circumstances. RMI is available to consult on this topic.
Modeling guidance:
- Calculate the EIR forward interest rate for the expected loan issuance year for the expected face value of loan term. Weight face value of loan term by Capex of assets to less than 30 years, if suitable.
- Choose an EIR leverage scenario or scenarios.
- For a given leverage scenario, calculate a WACC that includes EIR debt, corporate debt, and corporate equity. To reiterate, the EIR interest rate is fixed for the life of the loan.
- In capacity expansion modeling, apply WACC including EIR debt to eligible assets and WACC without EIR debt to ineligible assets. In some software (such as PLEXOS), this will be done by directly changing the cost of capital input. In others (such as GenX), modelers may need to implement this indirectly, through adjusting the annualized capital costs of candidate resources to account for lower cost of capital.
For EIR transaction costs, there is (per DOE guidance) a facility fee of 0.6% of project costs up to $2 billion, and 0.1% after that initial $2 billion in costs. There are also third-party expenses, which range from $1 to $3 million for projects financed by DOE under Title 17; RMI recommends use of the lower bound of $1 million, as due diligence for EIR projects likely to be far simpler than for non-EIR Title 17 projects, which can have innovative and emerging technologies. These should be treated as up-front costs. There is also an annual EIR maintenance fee ranging from $150,000 to $500,000, depending on the complexity; again, RMI recommends using the lower bound.
Modeling guidance:
- Add up-front transaction costs to EIR asset capital costs, proportional to asset cost.
- Add maintenance fees to operating expenses. In most capacity expansion software, it will be appropriate to input these fees as fixed operations and maintenance (O&M) costs.
Asset parameters
Assets eligible for EIR loans must be tagged to indicate whether the associated financing charges will be calculated with the inclusion of EIR debt or at the unsubsidized utility rate of return. EIR program guidance issued by DOE includes information about eligible project types. In addition, EIR asset costs may need to be adjusted to account for incremental programmatic compliance burdens.
EIR-funded projects must be compliant with the Davis-Bacon Act (i.e., they must pay prevailing wages), National Environmental Policy Act (NEPA), and the Cargo Preference Act. These compliance considerations are discussed at length in Maximizing the Value of the Energy Infrastructure Reinvestment Program for Utility Customers. We offer the following guidance for how to estimate the cost of compliance with these laws:
Davis-Bacon Act compliance costs: In many areas of the country, project cost estimates may already assume the payment of wages similar to those required under Davis-Bacon, especially if unionized labor is the norm. Where this is not the case, since no generally applicable rule can be offered, modelers should seek to establish whether assumed labor costs will differ significantly if EIR financing is employed.
For the weekly payroll requirement, if this capacity is currently lacking, RMI believes it appropriate to assume a single, up-front system upgrade cost that does not scale; once mechanisms are put in place to make one project compliant with weekly payroll processing, the incremental costs for subsequent projects should be negligible.
National Environmental Policy Act (NEPA) review costs: NEPA compliance costs and time delays are likely to be minimal if projects are of types “categorically excluded (CATEX).” In 2024, DOE expanded the categorical exclusions for solar photovoltaic systems on previously disturbed land and for upgrading and rebuilding transmission lines; it also created a categorical exclusion for some energy storage systems (including battery storage) on previously disturbed land.[16] However, some types of projects may trigger more onerous environmental assessments (EA) and Environmental Impact Statements (EIS). EAs typically takes 6 to 9 months, while the even more intensive EIS process, required for projects that will “significantly affect the quality of the human environment,” may take 1.5 to 2 years to complete. Current cost information for performing EA and EIS reviews is hard to come by. A 2014 study by the US Government Accountability Office (GAO) cited DOE data putting the median cost paid to contractors to perform an EIS review from 2003 to 2012 at $1.4 million.[17] Adjusting this figure for inflation from 2007 through 2024 would bring the median cost to $2.1 million. To reiterate, solar, transmission and storage projects on previously disturbed land will very likely qualify for categorical exclusion.[15] In reviewing modeling parameters, regulators should closely scrutinize EA or EIS treatment of asset types covered by an existing DOE CATEX.
Cargo Preference Act compliance costs: Compliance with Cargo Preference necessitates reliance on US-flagged vessels to transport cargo owned, procured, furnished, or financed by the US government. At this point, RMI cannot reasonably gauge the cost impact of the Cargo Preference Act, not least of all because demand for US-flagged shipping will be affected by the number of EIR projects undertaken (by individual utilities and nationwide). Once a baseline for EIR projects in a cost-optimal portfolio for a particular utility is determined, sensitivity analysis should be employed to test the impact of estimated Cargo Preference Act premiums.
Modeling guidance:
- Determine whether asset capex costs must be adjusted to reflect Davis-Bacon wages during construction. An adjustment may also be needed for maintenance capex where those are entered into the model.
- Regarding NEPA, solar and storage projects on previously disturbed land as well as transmission upgrades should, in the opinion of RMI, be treated as categorically excluded, resulting in negligible cost impacts and timing delays. For projects for which a categorical exclusion cannot be plausibly assumed, scenario analyses to account for EA and EIS cost and timing impacts would be reasonable; alternatively, these projects could be excluded from EIR financing treatment.
- For the Cargo Preference Act, RMI recommends modeling the EIR baseline without an explicit cost impact, followed by scenario analysis to test how potential incremental shipping costs might increase capex costs or affect assets and in turn reduce any PVRR advantage of an asset portfolio including EIR financing.
The EIR + Clean Repowering
Clean repowering savings potential will be highly site-specific. Modelers will need to estimate savings from reduced transmission interconnection costs while accounting for the associated operational constraints. A description of the four steps to modeling clean repowering in capacity expansion is available in the main text of this document; more detailed guidance is provided below.
Modeling guidance:
- Create site-specific opportunities for clean repowering resources at existing fossil fuel asset points of interconnection (POI). Wind, solar, and battery storage candidate resources should be made available at the POI, subject to physical and operational constraints.
- Physical constraints: RMI models the potential for unlimited battery storage connections and wind and solar connections capped at the capacities available within a given radius of existing fossil fuel assets, as per NREL’s solar and wind supply curve data. Other assumptions may be made to reflect on-the-ground project development realities known by the utility.
- Operational constraints: The combined output of existing thermal and new clean repowering resources should not exceed the maximum injection limit at the point of interconnection. Implementation of this constraint will vary across capacity expansion software. When possible, it should be done directly, by creating a joint constraint on hourly generation across the resources. It may also be possible to implement indirectly, by creating a new node (or equivalent) at the point of interconnection and instituting a transmission limit to the rest of the network with maximum flow equal to the point of interconnection injection limit. Note that the constraint should be placed on combined resource generation, not installed capacity, as the economically optimal solution to clean repowering may likely be to “overbuild” capacity behind the point of interconnection and use the hybrid resource flexibility to increase resource capacity factor. Enhanced availability from the hybrid resource is also likely to result in greater ability to meet peak demand, which should be reflected in the combined resource’s capacity accreditation.
- Reflect the value of clean repowering resources to mitigate interconnection delays. As described in the body of the main text, clean repowering resources are not subject to many of the interconnection delays faced by greenfield resources. In capacity expansion, the best way to model this is to remove any limits or constraints applied to annual capacity additions for clean repowering resources.
- Capture the economic benefits of clean repowering resources.
- One benefit of clean repowering resources is reduced transmission interconnection costs. In RMI’s modeling, these savings are captured by assuming the only transmission costs associated with the clean repowering resources are the costs of spur lines to connect to the existing thermal generation point of interconnection. Any other balance of system cost savings associated with developing hybrid rather than standalone resources should be reflected as well. All of these savings should be reflected in capacity expansion as reduced capital costs.
- Another economic benefit is the applicability of enhanced tax credits made available by the Inflation Reduction Act. In addition to the base value of the ITC or PTC, many clean repowering resources are likely to be eligible for the Energy Community Tax Credit Bonus given their proximity and intention connection to existing fossil infrastructure. This bonus credit provides up to an additional 10% of project costs if applied to the ITC or an additional 10% of the credit supplied by the PTC. Eligibility maps can be found on the program website. These savings should be modeled in capacity expansion as adjustments to resource capital costs.
- Finally, all clean repowering resources are eligible for EIR financing. These savings should be represented in capacity expansion the same as for other clean assets.
Endnotes
[1] E. Larson, C. Greig, J. Jenkins, E. Mayfield, A. Pascale, C. Zhang, J. Drossman, R. Williams, S. Pacala, R. Socolow, EJ Baik, R. Birdsey, R. Duke, R. Jones, B. Haley, E. Leslie, K. Paustian, and A. Swan, Net-Zero America: Potential Pathways, Infrastructure, and Impacts, Interim Report, Princeton University, 2020, https://netzeroamerica.princeton.edu/the-report.
[2] Support for this assumption is presented in Christian Fong, David Posner, and Uday Varadarajan, “Maximizing the Value of the Energy Infrastructure Reinvestment Program for Utility Customers,” RMI, May 24, 2024, https://rmi.org/maximizing-the-value-of-the-energy-infrastructure-reinvestment-program-for-utility-customers/.
[3] Docket No. E-100, Sub 190, “Testimony of Jeff Thomas Public Staff,” North Carolina Utilities Commission, May 29, 2024, https://starw1.ncuc.gov/NCUC/ViewFile.aspx?Id=eb84ecb4-3891-40a2-a7eb-426b153f1f3a.
[4] According to the US Department of Energy’s Title 17 Loan Program Guidance, to be eligible for EIR funding, investments need only “avoid, reduce, utilize, or sequester air pollutants and anthropogenic emissions of greenhouse gases,” which can include investments in generation resources, as well as a broad array of other technologies such as repowering or retooling energy infrastructure to operate more efficiently or at higher output. However, for the purposes of capacity expansion modeling, we recommend modelers at a minimum assume that all resources that do not emit greenhouse gases be defined as eligible for EIR funding within the model.
[5] Most clean resources selected by a capacity expansion model will likely qualify as “enabling existing energy infrastructure to reduce emissions,” but if these resources differ geographically from the location of existing infrastructure, more effort may be required to ensure compliance with EIR program eligibility requirements. The LPO website stipulates that EIR projects “must show a clear relationship between new services and benefits provided by the Title 17 financed infrastructure and services, and benefits lost from the legacy infrastructure that ceased operations, such as grid capacity, reliability, and workforce retention and opportunities, including if the replacement plan differs from the legacy infrastructure physically and/or geographically.” See https://www.energy.gov/lpo/energy-infrastructure-reinvestment.
[6] Docket U-240013, “Policy Statement Addressing the Federal Inflation Reduction Act and the Infrastructure Investment and Jobs Act in Utility Planning,” Washington Utilities and Transportation Commission, May 3, 2024, https://apiproxy.utc.wa.gov/cases/GetDocument?docID=31&year=2024&docketNumber=240013.
[7] Clean Repowering: How to Capitalize on Fossil Grid Connections to Unlock Clean Energy Growth, RMI, 2024, https://rmi.org/insight/clean-repowering/.
[8] Katie Siegner and Alex Engel, “Clean Repowering: A Near-Term, IRA-Powered Energy Transition Accelerant,” RMI January 16, 2024, https://rmi.org/clean-repowering-a-near-term-ira-powered-energy-transition-accelerant/.
[9] The cost and benefit calculation for project delays is a complex discounting equation. All else equal, delaying deployment should reduce the present value of costs due to discounting. However, there can be cost increases associated with construction financing loan extension, or other payments required for project delays. Additionally, we assume cost of deploying clean energy will decrease as time progresses, but it is uncertain whether the cost savings of postponed deployment will exceed the known savings associated with the EIR program, under which funds are available to be committed between now and September 2026 for projects (or project milestones) that must be completed by September 31, 2031.
[10] “Grid connection backlog grows by 30% in 2023, dominated by requests for solar, wind, and energy storage,” Berkely Lab, April 10, 2024, https://emp.lbl.gov/news/grid-connection-backlog-grows-30-2023-dominated-requests-solar-wind-and-energy-storage.
[11] The Synapse report “No-Regrets Solutions for Accelerating Grid Interconnection How Fast-Track Interconnection Processes Cut Costs in SPP and MISO” provides a real world example of the interconnection cost savings that can be achieved through clean repowering. A solar project in Sumner County, Kansas, had total interconnection costs before construction of approximately $333/kW, of which roughly 70% were for transmission network upgrades, with a wait of more than eight years to interconnect. In contrast, a solar project in Harper County, Kansas, used surplus interconnection, and there were no transmission network upgrades associated with this project, resulting in less than $1/kW total interconnection costs before construction.
[12] RMI’s article “For Ratepayers to Realize Savings from Clean Energy, Utility Business Models Need an Update” elaborates on the ways in which taking advantage of IRA opportunities is misaligned with traditional utility incentives.
[13] As described in documents from the 2021 NARUC-NASEO Comprehensive Electricity Planning Framework Taskforce and 2021 FERC Joint Federal-State Task Force on Electric Transmission. For further discussion and examples of leading practices, see Mark Dyson, Lauren Shwisberg, and Katerina Stephan, Reimagining Resource Planning, RMI, January 2023, https://rmi.org/insight/reimagining-resource-planning/.
[14] Christian Fong, David Posner, and Uday Varadarajan, “The Energy Infrastructure Reinvestment Program: Federal financing for an equitable, clean economy,” RMI, February 16, 2024, https://rmi.org/the-energy-infrastructure-reinvestment-program-federal-financing-for-an-equitable-clean-economy/.
[15] The 2014 GAO report also noted that 95% of DOE’s 9,060 NEPA analyses from 2008 to 2012 were categorical exclusions; ibid., 7.
[16] DOE NEPA Categorical Exclusion Rulemaking, DOE, 2024, https://www.energy.gov/sites/default/files/2024-04/doe-10-cfr-1021-final-rule-2024-04-30-final.pdf.
[17] National Environmental Policy Act: Little Information Exists on NEPA Analyses, GAO-14-369, April 15, 2014, https://www.gao.gov/products/gao-14-370.
[18] LPO’s Energy Infrastructure Reinvestment (1706) Program https://www.energy.gov/lpo/articles/lpos-energy-infrastructure-reinvestment-1706-program
Transmission system investment may be eligible for EIR financing, subject to LPO review of the potential to reduce systemwide greenhouse gas emissions. Eligible investment may include reconductoring of existing lines, deployment of grid-enhancing technologies (GETs), and new transmission infrastructure if it can be linked to enabling the reduction of GHG emissions.
Utilities and their customers generally stand to benefit from including any planned transmission investments in their EIR applications. If the utilities can make the case that these transmission investments facilitate clean energy integration, the cost of developing already-planned transmission investments could be lower.
To maximize customer savings, utilities can consider opportunities to leverage EIR funding to support more ambitious transmission plans that in turn facilitate the integration of additional clean energy generation. Because both the transmission upgrades and clean energy additions are eligible for EIR financing, accelerated deployment plans will be more economically competitive than ever. Depending on data availability, utilities can conduct this analysis in one of the two following ways.
Option 3.a: The first method is to co-optimize transmission investment and generation capacity expansion. This can be done if the utility is able to parametrize the cost of transmission upgrades and implement them directly into generation capacity expansion software. Planners can modify the costs of both transmission investments and clean generation capacity to account for savings from EIR financing, and then let their models determine the optimal portfolio of EIR-financed transmission, EIR-financed clean generation, and utility-financed conventional generation.
Option 3.b: The second method is to test the impacts of utilizing different transmission investment plans as inputs to the generation capacity expansion model. This approach may be more suitable for utilities that conduct transmission and generation capacity expansion planning separately. In this situation, it is worthwhile for planners to test whether access to EIR financing enables a more aggressive transmission investment plan than would otherwise be economic.
Exhibits 3 and 4 below show a stylized example of how EIR financing could alter the optimal transmission investment pathway, in keeping with the second method (Option 3.b) described above. In this example, the utility has modeled two transmission pathways: “Base Transmission (1,000 MW)” and “Aggressive Transmission (1,500 MW).”
Exhibit 3 compares capacity expansion outputs across the two transmission pathways, utilizing utility-only financing.
- Using the Base Transmission case as an input, the capacity expansion model selects 8,000 MW of fossil generation and 2,000 MW of clean generation as the cost-optimal generation portfolio. The total cost of this portfolio is $2,400 million per year, of which $2,200 million is for generation and $200 million for transmission.
- Using the Aggressive Transmission investment plan as an input, the cost-optimal generation portfolio changes: it now comprises 7,000 MW of fossil generation and 4,000 MW of clean generation. Generation costs are $2,050 million per year. Accounting for transmission costs of $400 million per year, the cost of this portfolio is $2,450 million per year.
While the Aggressive Transmission case enables a lower-cost generation capacity expansion plan, total (generation plus transmission) cost is lower under the Base Transmission plan. Therefore, we can expect planners to pick the Base Transmission plan and accompanying fossil-heavy generation mix as the preferred resource portfolio.
Exhibit 4 compares capacity expansion outputs across the two transmission pathways once more, but this time we consider the impacts of including EIR financing on transmission and clean energy expansion. We assume that transmission has an economic lifetime of 40 years, clean resources have an economic lifetime of 20 years, and EIR financing lowers the after-tax financing costs for both types of eligible resources from 10% to 6%. For simplicity, we also assume the total MW buildout of each resource is the same as in the no-EIR financing situation.
Again, we see that the optimal generation portfolio under the Aggressive Transmission case is cleaner and the generation components less costly than under the Base Transmission case. Transmission costs remain larger under the Aggressive Transmission case than under the Base Transmission case. However, with EIR financing, the all-in cost picture has flipped: the Aggressive Transmission pathway, with its greater levels of clean energy integration, is now the lowest-cost option. Taking EIR into account, we can expect planners to advance the Aggressive Transmission case with the cleaner generation mix as their preferred portfolio.
Recommendations for Regulators:
Integrated transmission and generation planning is the gold standard of utility planning.[15] Yet, in many cases, coordination between transmission and generation planning remains limited. Failing to consider how transmission and generation may interact with respect to EIR is likely to lead to an underestimation of EIR-eligible investment, leaving savings on the table. To maximize EIR benefits across transmission and generation, regulators can:
- Request that utilities conduct analysis of planned and potential transmission upgrades (including reconductoring and GETs) as well as additional new lines that have the potential to support greater levels of new resources eligible for the EIR, reporting these findings to the Commission prior to submission of an EIR application; and
- Encourage utilities to model the impacts of the EIR on enhanced transmission investment portfolios, either through co-optimizing transmission investment and generation expansion or through scenario-based analysis.
LPO needs at least 6 months and often upward of a year to review applications for funding. Realistically, that means utilities need to apply for EIR funding by the end of 2025 to meet the September 30, 2026, conditional commitment date. But it is critical to recognize that LPO is willing to consider EIR funding for projects which, at the time of application, are at different stages of regulatory consideration and development. To quote from a recent LPO announcement on this topic, applications may:
include a combination of identified near-term projects and less defined, longer-term projects associated with an IRP in a single loan application. Utilities don’t need to wait for regulatory approval to submit an application. Regulatory approvals can occur during the due diligence phase or can be included as conditions precedent or covenants in the loan.2
This LPO guidance underscores the importance of integrated resource planning (IRP) efforts over the next year, in time to inform EIR applications.
The resource planning process is where system needs and economic parameters are optimized. The composition of a least-cost resource portfolio is likely to be significantly affected by the EIR eligibility of the proposed assets as well as the share of EIR debt (i.e., the leverage) assumed for their capital stacks. These factors can be assessed via capacity expansion modeling that is customary in IRP proceedings.
Conclusion
Integrated resource planning is an unparalleled opportunity for utilities, regulators, and stakeholders to prepare the electricity system for future opportunities and risks while also delivering cost-effective service.
RMI has previously called the EIR the “most important clean energy policy you’ve never heard about,” because of its potential to revitalize local communities historically dependent on fossil fuel infrastructure while saving electricity ratepayers money and building new clean energy resources — a triple win for communities, customers, and the climate.[16]
Given the significance of the resource planning process and the time-limited cost-savings potential of the EIR, the two must be mutually informing during the next 12 months. We hope that this insight brief supports utility planners and regulators alike in assessing the maximum savings potential that the EIR can offer their service territories and submitting competitive applications to deliver the savings to ratepayers.
Technical Appendix: Detailed EIR Modeling Instructions for Utility Planners
In the insight brief above, we offer a high-level conceptual approach to incorporating the EIR into a modeling process. This Technical Appendix provides more granular recommendations for how to implement this approach within capacity expansion modeling software.
EIR financing must be reflected in capacity expansion modeling both within the financing parameters and the asset parameters. The purpose of this Technical Appendix is to support utility planners and modelers endeavoring to incorporate the appropriate financing and asset parameters into a modeling software of their choice. Because each modeling software is slightly different, we offer specific yet software-neutral recommendations for implementation. RMI is available for consultation if more explicit modeling guidance is necessary.
Financing parameters
EIR debt is fixed interest rate lending with a maximum term allowed under the law of 30 years, consistent with the forecasted operating life of many utility assets. For some assets (e.g., battery storage), shorter loan terms may be appropriate. Especially long-lived assets (e.g., transmission) should account for a refinancing without EIR financing after 30 years. Thanks to its $5 billion credit subsidy appropriation, DOE is able to offer loans at interest rates as low as 37.5 basis points above the federal government’s cost of borrowing for a similar duration.
Bootstrapping of current Treasury Yield Curves can be used to estimate a forward rate for the appropriate loan term. RMI is available to assist with this calculation, which is laid out below:
- Fit a quadratic equation (ax2 + bx + c) to the Daily Treasury Par Yield Curve Rates, available from the US Department of the Treasury.
- All equations below must be solved iteratively.
- Using the coefficients from the quadratic equation from Step i, calculate:
- Spot rate at issuance = (((Year of Issuance – Year of Yield Curve)2)*a + (Year of Issuance – Year of Yield Curve)*b + c)/100
- Spot rate at Maturity = (((Year of Issuance + Weighted Average Tenor of Loan – Year of Yield Curve)2)*a + (Year of Issuance + Weighted Average Tenor of Loan – Year of Yield Curve)*b + c)/100
- Forward Treasury Rate for Loan = ((1 + Spot Rate at Maturity)^(Year of Issuance + Weighted Average Tenor of Loan – Year of Yield Curve)/(1 + Spot Rate at Issuance )^(Year of Issuance – Year of Yield Curve))^(1/Weighted Average Tenor of Loan) – 1
- Forward EIR Loan Rate = Forward Treasury Rate + 37.5 basis points
- Weighted Average Tenor of Loan = (Face Tenor of Loan +1) – (1+Iterated Value of Forward EIR Loan Rate)/Iterated Value of Forward EIR Loan Rate + Face Tenor of Loan/((1 + Iterated Value of Forward EIR Loan)^(Face Tenor of Loan) – 1)
DOE may make EIR loans for up to 80% of the value of eligible projects, with the balance being provided by the borrower. A combination of 80% EIR lending and 20% utility financing will result in a far lower weighted average cost of capital (WACC) than normal utility financing, which typically combines shareholder equity and corporate debt in roughly equal shares. Critically, EIR projects need to be evaluated for total ratepayer cost using a cost of capital that is distinct from the rate of return applied to non-EIR projects.
RMI recommends that high-leverage EIR loans be structured as off-balance sheet financings without recourse to the utility’s balance sheet in order to insulate the company’s other debt and equity from credit negative cost impacts. Sensitivity testing that accounts for feedback of on-balance sheet EIR lending on the utility’s other financing costs may be appropriate in certain circumstances. RMI is available to consult on this topic.
Modeling guidance:
- Calculate the EIR forward interest rate for the expected loan issuance year for the expected face value of loan term. Weight face value of loan term by Capex of assets to less than 30 years, if suitable.
- Choose an EIR leverage scenario or scenarios.
- For a given leverage scenario, calculate a WACC that includes EIR debt, corporate debt, and corporate equity. To reiterate, the EIR interest rate is fixed for the life of the loan.
- In capacity expansion modeling, apply WACC including EIR debt to eligible assets and WACC without EIR debt to ineligible assets. In some software (such as PLEXOS), this will be done by directly changing the cost of capital input. In others (such as GenX), modelers may need to implement this indirectly, through adjusting the annualized capital costs of candidate resources to account for lower cost of capital.
For EIR transaction costs, there is (per DOE guidance) a facility fee of 0.6% of project costs up to $2 billion, and 0.1% after that initial $2 billion in costs. There are also third-party expenses, which range from $1 to $3 million for projects financed by DOE under Title 17; RMI recommends use of the lower bound of $1 million, as due diligence for EIR projects likely to be far simpler than for non-EIR Title 17 projects, which can have innovative and emerging technologies. These should be treated as up-front costs. There is also an annual EIR maintenance fee ranging from $150,000 to $500,000, depending on the complexity; again, RMI recommends using the lower bound.
Modeling guidance:
- Add up-front transaction costs to EIR asset capital costs, proportional to asset cost.
- Add maintenance fees to operating expenses. In most capacity expansion software, it will be appropriate to input these fees as fixed operations and maintenance (O&M) costs.
Asset parameters
Assets eligible for EIR loans must be tagged to indicate whether the associated financing charges will be calculated with the inclusion of EIR debt or at the unsubsidized utility rate of return. EIR program guidance issued by DOE includes information about eligible project types. In addition, EIR asset costs may need to be adjusted to account for incremental programmatic compliance burdens.
EIR-funded projects must be compliant with the Davis-Bacon Act (i.e., they must pay prevailing wages), National Environmental Policy Act (NEPA), and the Cargo Preference Act. These compliance considerations are discussed at length in Maximizing the Value of the Energy Infrastructure Reinvestment Program for Utility Customers. We offer the following guidance for how to estimate the cost of compliance with these laws:
Davis-Bacon Act compliance costs: In many areas of the country, project cost estimates may already assume the payment of wages similar to those required under Davis-Bacon, especially if unionized labor is the norm. Where this is not the case, since no generally applicable rule can be offered, modelers should seek to establish whether assumed labor costs will differ significantly if EIR financing is employed.
For the weekly payroll requirement, if this capacity is currently lacking, RMI believes it appropriate to assume a single, up-front system upgrade cost that does not scale; once mechanisms are put in place to make one project compliant with weekly payroll processing, the incremental costs for subsequent projects should be negligible.
National Environmental Policy Act (NEPA) review costs: NEPA compliance costs and time delays are likely to be minimal if projects are of types “categorically excluded (CATEX).” In 2024, DOE expanded the categorical exclusions for solar photovoltaic systems on previously disturbed land and for upgrading and rebuilding transmission lines; it also created a categorical exclusion for some energy storage systems (including battery storage) on previously disturbed land.[16] However, some types of projects may trigger more onerous environmental assessments (EA) and Environmental Impact Statements (EIS). EAs typically takes 6 to 9 months, while the even more intensive EIS process, required for projects that will “significantly affect the quality of the human environment,” may take 1.5 to 2 years to complete. Current cost information for performing EA and EIS reviews is hard to come by. A 2014 study by the US Government Accountability Office (GAO) cited DOE data putting the median cost paid to contractors to perform an EIS review from 2003 to 2012 at $1.4 million.[17] Adjusting this figure for inflation from 2007 through 2024 would bring the median cost to $2.1 million. To reiterate, solar, transmission and storage projects on previously disturbed land will very likely qualify for categorical exclusion.[15] In reviewing modeling parameters, regulators should closely scrutinize EA or EIS treatment of asset types covered by an existing DOE CATEX.
Cargo Preference Act compliance costs: Compliance with Cargo Preference necessitates reliance on US-flagged vessels to transport cargo owned, procured, furnished, or financed by the US government. At this point, RMI cannot reasonably gauge the cost impact of the Cargo Preference Act, not least of all because demand for US-flagged shipping will be affected by the number of EIR projects undertaken (by individual utilities and nationwide). Once a baseline for EIR projects in a cost-optimal portfolio for a particular utility is determined, sensitivity analysis should be employed to test the impact of estimated Cargo Preference Act premiums.
Modeling guidance:
- Determine whether asset capex costs must be adjusted to reflect Davis-Bacon wages during construction. An adjustment may also be needed for maintenance capex where those are entered into the model.
- Regarding NEPA, solar and storage projects on previously disturbed land as well as transmission upgrades should, in the opinion of RMI, be treated as categorically excluded, resulting in negligible cost impacts and timing delays. For projects for which a categorical exclusion cannot be plausibly assumed, scenario analyses to account for EA and EIS cost and timing impacts would be reasonable; alternatively, these projects could be excluded from EIR financing treatment.
- For the Cargo Preference Act, RMI recommends modeling the EIR baseline without an explicit cost impact, followed by scenario analysis to test how potential incremental shipping costs might increase capex costs or affect assets and in turn reduce any PVRR advantage of an asset portfolio including EIR financing.
The EIR + Clean Repowering
Clean repowering savings potential will be highly site-specific. Modelers will need to estimate savings from reduced transmission interconnection costs while accounting for the associated operational constraints. A description of the four steps to modeling clean repowering in capacity expansion is available in the main text of this document; more detailed guidance is provided below.
Modeling guidance:
- Create site-specific opportunities for clean repowering resources at existing fossil fuel asset points of interconnection (POI). Wind, solar, and battery storage candidate resources should be made available at the POI, subject to physical and operational constraints.
- Physical constraints: RMI models the potential for unlimited battery storage connections and wind and solar connections capped at the capacities available within a given radius of existing fossil fuel assets, as per NREL’s solar and wind supply curve data. Other assumptions may be made to reflect on-the-ground project development realities known by the utility.
- Operational constraints: The combined output of existing thermal and new clean repowering resources should not exceed the maximum injection limit at the point of interconnection. Implementation of this constraint will vary across capacity expansion software. When possible, it should be done directly, by creating a joint constraint on hourly generation across the resources. It may also be possible to implement indirectly, by creating a new node (or equivalent) at the point of interconnection and instituting a transmission limit to the rest of the network with maximum flow equal to the point of interconnection injection limit. Note that the constraint should be placed on combined resource generation, not installed capacity, as the economically optimal solution to clean repowering may likely be to “overbuild” capacity behind the point of interconnection and use the hybrid resource flexibility to increase resource capacity factor. Enhanced availability from the hybrid resource is also likely to result in greater ability to meet peak demand, which should be reflected in the combined resource’s capacity accreditation.
- Reflect the value of clean repowering resources to mitigate interconnection delays. As described in the body of the main text, clean repowering resources are not subject to many of the interconnection delays faced by greenfield resources. In capacity expansion, the best way to model this is to remove any limits or constraints applied to annual capacity additions for clean repowering resources.
- Capture the economic benefits of clean repowering resources.
- One benefit of clean repowering resources is reduced transmission interconnection costs. In RMI’s modeling, these savings are captured by assuming the only transmission costs associated with the clean repowering resources are the costs of spur lines to connect to the existing thermal generation point of interconnection. Any other balance of system cost savings associated with developing hybrid rather than standalone resources should be reflected as well. All of these savings should be reflected in capacity expansion as reduced capital costs.
- Another economic benefit is the applicability of enhanced tax credits made available by the Inflation Reduction Act. In addition to the base value of the ITC or PTC, many clean repowering resources are likely to be eligible for the Energy Community Tax Credit Bonus given their proximity and intention connection to existing fossil infrastructure. This bonus credit provides up to an additional 10% of project costs if applied to the ITC or an additional 10% of the credit supplied by the PTC. Eligibility maps can be found on the program website. These savings should be modeled in capacity expansion as adjustments to resource capital costs.
- Finally, all clean repowering resources are eligible for EIR financing. These savings should be represented in capacity expansion the same as for other clean assets.
Endnotes
[1] E. Larson, C. Greig, J. Jenkins, E. Mayfield, A. Pascale, C. Zhang, J. Drossman, R. Williams, S. Pacala, R. Socolow, EJ Baik, R. Birdsey, R. Duke, R. Jones, B. Haley, E. Leslie, K. Paustian, and A. Swan, Net-Zero America: Potential Pathways, Infrastructure, and Impacts, Interim Report, Princeton University, 2020, https://netzeroamerica.princeton.edu/the-report.
[2] Support for this assumption is presented in Christian Fong, David Posner, and Uday Varadarajan, “Maximizing the Value of the Energy Infrastructure Reinvestment Program for Utility Customers,” RMI, May 24, 2024, https://rmi.org/maximizing-the-value-of-the-energy-infrastructure-reinvestment-program-for-utility-customers/.
[3] Docket No. E-100, Sub 190, “Testimony of Jeff Thomas Public Staff,” North Carolina Utilities Commission, May 29, 2024, https://starw1.ncuc.gov/NCUC/ViewFile.aspx?Id=eb84ecb4-3891-40a2-a7eb-426b153f1f3a.
[4] According to the US Department of Energy’s Title 17 Loan Program Guidance, to be eligible for EIR funding, investments need only “avoid, reduce, utilize, or sequester air pollutants and anthropogenic emissions of greenhouse gases,” which can include investments in generation resources, as well as a broad array of other technologies such as repowering or retooling energy infrastructure to operate more efficiently or at higher output. However, for the purposes of capacity expansion modeling, we recommend modelers at a minimum assume that all resources that do not emit greenhouse gases be defined as eligible for EIR funding within the model.
[5] Most clean resources selected by a capacity expansion model will likely qualify as “enabling existing energy infrastructure to reduce emissions,” but if these resources differ geographically from the location of existing infrastructure, more effort may be required to ensure compliance with EIR program eligibility requirements. The LPO website stipulates that EIR projects “must show a clear relationship between new services and benefits provided by the Title 17 financed infrastructure and services, and benefits lost from the legacy infrastructure that ceased operations, such as grid capacity, reliability, and workforce retention and opportunities, including if the replacement plan differs from the legacy infrastructure physically and/or geographically.” See https://www.energy.gov/lpo/energy-infrastructure-reinvestment.
[6] Docket U-240013, “Policy Statement Addressing the Federal Inflation Reduction Act and the Infrastructure Investment and Jobs Act in Utility Planning,” Washington Utilities and Transportation Commission, May 3, 2024, https://apiproxy.utc.wa.gov/cases/GetDocument?docID=31&year=2024&docketNumber=240013.
[7] Clean Repowering: How to Capitalize on Fossil Grid Connections to Unlock Clean Energy Growth, RMI, 2024, https://rmi.org/insight/clean-repowering/.
[8] Katie Siegner and Alex Engel, “Clean Repowering: A Near-Term, IRA-Powered Energy Transition Accelerant,” RMI January 16, 2024, https://rmi.org/clean-repowering-a-near-term-ira-powered-energy-transition-accelerant/.
[9] The cost and benefit calculation for project delays is a complex discounting equation. All else equal, delaying deployment should reduce the present value of costs due to discounting. However, there can be cost increases associated with construction financing loan extension, or other payments required for project delays. Additionally, we assume cost of deploying clean energy will decrease as time progresses, but it is uncertain whether the cost savings of postponed deployment will exceed the known savings associated with the EIR program, under which funds are available to be committed between now and September 2026 for projects (or project milestones) that must be completed by September 31, 2031.
[10] “Grid connection backlog grows by 30% in 2023, dominated by requests for solar, wind, and energy storage,” Berkely Lab, April 10, 2024, https://emp.lbl.gov/news/grid-connection-backlog-grows-30-2023-dominated-requests-solar-wind-and-energy-storage.
[11] The Synapse report “No-Regrets Solutions for Accelerating Grid Interconnection How Fast-Track Interconnection Processes Cut Costs in SPP and MISO” provides a real world example of the interconnection cost savings that can be achieved through clean repowering. A solar project in Sumner County, Kansas, had total interconnection costs before construction of approximately $333/kW, of which roughly 70% were for transmission network upgrades, with a wait of more than eight years to interconnect. In contrast, a solar project in Harper County, Kansas, used surplus interconnection, and there were no transmission network upgrades associated with this project, resulting in less than $1/kW total interconnection costs before construction.
[12] RMI’s article “For Ratepayers to Realize Savings from Clean Energy, Utility Business Models Need an Update” elaborates on the ways in which taking advantage of IRA opportunities is misaligned with traditional utility incentives.
[13] As described in documents from the 2021 NARUC-NASEO Comprehensive Electricity Planning Framework Taskforce and 2021 FERC Joint Federal-State Task Force on Electric Transmission. For further discussion and examples of leading practices, see Mark Dyson, Lauren Shwisberg, and Katerina Stephan, Reimagining Resource Planning, RMI, January 2023, https://rmi.org/insight/reimagining-resource-planning/.
[14] Christian Fong, David Posner, and Uday Varadarajan, “The Energy Infrastructure Reinvestment Program: Federal financing for an equitable, clean economy,” RMI, February 16, 2024, https://rmi.org/the-energy-infrastructure-reinvestment-program-federal-financing-for-an-equitable-clean-economy/.
[15] The 2014 GAO report also noted that 95% of DOE’s 9,060 NEPA analyses from 2008 to 2012 were categorical exclusions; ibid., 7.
[16] DOE NEPA Categorical Exclusion Rulemaking, DOE, 2024, https://www.energy.gov/sites/default/files/2024-04/doe-10-cfr-1021-final-rule-2024-04-30-final.pdf.
[17] National Environmental Policy Act: Little Information Exists on NEPA Analyses, GAO-14-369, April 15, 2014, https://www.gao.gov/products/gao-14-370.
[18] LPO’s Energy Infrastructure Reinvestment (1706) Program https://www.energy.gov/lpo/articles/lpos-energy-infrastructure-reinvestment-1706-program
Integrated resource planning is an unparalleled opportunity for utilities, regulators, and stakeholders to prepare the electricity system for future opportunities and risks while also delivering cost-effective service.
RMI has previously called the EIR the “most important clean energy policy you’ve never heard about,” because of its potential to revitalize local communities historically dependent on fossil fuel infrastructure while saving electricity ratepayers money and building new clean energy resources — a triple win for communities, customers, and the climate.[16]
Given the significance of the resource planning process and the time-limited cost-savings potential of the EIR, the two must be mutually informing during the next 12 months. We hope that this insight brief supports utility planners and regulators alike in assessing the maximum savings potential that the EIR can offer their service territories and submitting competitive applications to deliver the savings to ratepayers.
In the insight brief above, we offer a high-level conceptual approach to incorporating the EIR into a modeling process. This Technical Appendix provides more granular recommendations for how to implement this approach within capacity expansion modeling software.
EIR financing must be reflected in capacity expansion modeling both within the financing parameters and the asset parameters. The purpose of this Technical Appendix is to support utility planners and modelers endeavoring to incorporate the appropriate financing and asset parameters into a modeling software of their choice. Because each modeling software is slightly different, we offer specific yet software-neutral recommendations for implementation. RMI is available for consultation if more explicit modeling guidance is necessary.
Financing parameters
EIR debt is fixed interest rate lending with a maximum term allowed under the law of 30 years, consistent with the forecasted operating life of many utility assets. For some assets (e.g., battery storage), shorter loan terms may be appropriate. Especially long-lived assets (e.g., transmission) should account for a refinancing without EIR financing after 30 years. Thanks to its $5 billion credit subsidy appropriation, DOE is able to offer loans at interest rates as low as 37.5 basis points above the federal government’s cost of borrowing for a similar duration.
Bootstrapping of current Treasury Yield Curves can be used to estimate a forward rate for the appropriate loan term. RMI is available to assist with this calculation, which is laid out below:
- Fit a quadratic equation (ax2 + bx + c) to the Daily Treasury Par Yield Curve Rates, available from the US Department of the Treasury.
- All equations below must be solved iteratively.
- Using the coefficients from the quadratic equation from Step i, calculate:
- Spot rate at issuance = (((Year of Issuance – Year of Yield Curve)2)*a + (Year of Issuance – Year of Yield Curve)*b + c)/100
- Spot rate at Maturity = (((Year of Issuance + Weighted Average Tenor of Loan – Year of Yield Curve)2)*a + (Year of Issuance + Weighted Average Tenor of Loan – Year of Yield Curve)*b + c)/100
- Forward Treasury Rate for Loan = ((1 + Spot Rate at Maturity)^(Year of Issuance + Weighted Average Tenor of Loan – Year of Yield Curve)/(1 + Spot Rate at Issuance )^(Year of Issuance – Year of Yield Curve))^(1/Weighted Average Tenor of Loan) – 1
- Forward EIR Loan Rate = Forward Treasury Rate + 37.5 basis points
- Weighted Average Tenor of Loan = (Face Tenor of Loan +1) – (1+Iterated Value of Forward EIR Loan Rate)/Iterated Value of Forward EIR Loan Rate + Face Tenor of Loan/((1 + Iterated Value of Forward EIR Loan)^(Face Tenor of Loan) – 1)
DOE may make EIR loans for up to 80% of the value of eligible projects, with the balance being provided by the borrower. A combination of 80% EIR lending and 20% utility financing will result in a far lower weighted average cost of capital (WACC) than normal utility financing, which typically combines shareholder equity and corporate debt in roughly equal shares. Critically, EIR projects need to be evaluated for total ratepayer cost using a cost of capital that is distinct from the rate of return applied to non-EIR projects.
RMI recommends that high-leverage EIR loans be structured as off-balance sheet financings without recourse to the utility’s balance sheet in order to insulate the company’s other debt and equity from credit negative cost impacts. Sensitivity testing that accounts for feedback of on-balance sheet EIR lending on the utility’s other financing costs may be appropriate in certain circumstances. RMI is available to consult on this topic.
Modeling guidance:
- Calculate the EIR forward interest rate for the expected loan issuance year for the expected face value of loan term. Weight face value of loan term by Capex of assets to less than 30 years, if suitable.
- Choose an EIR leverage scenario or scenarios.
- For a given leverage scenario, calculate a WACC that includes EIR debt, corporate debt, and corporate equity. To reiterate, the EIR interest rate is fixed for the life of the loan.
- In capacity expansion modeling, apply WACC including EIR debt to eligible assets and WACC without EIR debt to ineligible assets. In some software (such as PLEXOS), this will be done by directly changing the cost of capital input. In others (such as GenX), modelers may need to implement this indirectly, through adjusting the annualized capital costs of candidate resources to account for lower cost of capital.
For EIR transaction costs, there is (per DOE guidance) a facility fee of 0.6% of project costs up to $2 billion, and 0.1% after that initial $2 billion in costs. There are also third-party expenses, which range from $1 to $3 million for projects financed by DOE under Title 17; RMI recommends use of the lower bound of $1 million, as due diligence for EIR projects likely to be far simpler than for non-EIR Title 17 projects, which can have innovative and emerging technologies. These should be treated as up-front costs. There is also an annual EIR maintenance fee ranging from $150,000 to $500,000, depending on the complexity; again, RMI recommends using the lower bound.
Modeling guidance:
- Add up-front transaction costs to EIR asset capital costs, proportional to asset cost.
- Add maintenance fees to operating expenses. In most capacity expansion software, it will be appropriate to input these fees as fixed operations and maintenance (O&M) costs.
Asset parameters
Assets eligible for EIR loans must be tagged to indicate whether the associated financing charges will be calculated with the inclusion of EIR debt or at the unsubsidized utility rate of return. EIR program guidance issued by DOE includes information about eligible project types. In addition, EIR asset costs may need to be adjusted to account for incremental programmatic compliance burdens.
EIR-funded projects must be compliant with the Davis-Bacon Act (i.e., they must pay prevailing wages), National Environmental Policy Act (NEPA), and the Cargo Preference Act. These compliance considerations are discussed at length in Maximizing the Value of the Energy Infrastructure Reinvestment Program for Utility Customers. We offer the following guidance for how to estimate the cost of compliance with these laws:
Davis-Bacon Act compliance costs: In many areas of the country, project cost estimates may already assume the payment of wages similar to those required under Davis-Bacon, especially if unionized labor is the norm. Where this is not the case, since no generally applicable rule can be offered, modelers should seek to establish whether assumed labor costs will differ significantly if EIR financing is employed.
For the weekly payroll requirement, if this capacity is currently lacking, RMI believes it appropriate to assume a single, up-front system upgrade cost that does not scale; once mechanisms are put in place to make one project compliant with weekly payroll processing, the incremental costs for subsequent projects should be negligible.
National Environmental Policy Act (NEPA) review costs: NEPA compliance costs and time delays are likely to be minimal if projects are of types “categorically excluded (CATEX).” In 2024, DOE expanded the categorical exclusions for solar photovoltaic systems on previously disturbed land and for upgrading and rebuilding transmission lines; it also created a categorical exclusion for some energy storage systems (including battery storage) on previously disturbed land.[16] However, some types of projects may trigger more onerous environmental assessments (EA) and Environmental Impact Statements (EIS). EAs typically takes 6 to 9 months, while the even more intensive EIS process, required for projects that will “significantly affect the quality of the human environment,” may take 1.5 to 2 years to complete. Current cost information for performing EA and EIS reviews is hard to come by. A 2014 study by the US Government Accountability Office (GAO) cited DOE data putting the median cost paid to contractors to perform an EIS review from 2003 to 2012 at $1.4 million.[17] Adjusting this figure for inflation from 2007 through 2024 would bring the median cost to $2.1 million. To reiterate, solar, transmission and storage projects on previously disturbed land will very likely qualify for categorical exclusion.[15] In reviewing modeling parameters, regulators should closely scrutinize EA or EIS treatment of asset types covered by an existing DOE CATEX.
Cargo Preference Act compliance costs: Compliance with Cargo Preference necessitates reliance on US-flagged vessels to transport cargo owned, procured, furnished, or financed by the US government. At this point, RMI cannot reasonably gauge the cost impact of the Cargo Preference Act, not least of all because demand for US-flagged shipping will be affected by the number of EIR projects undertaken (by individual utilities and nationwide). Once a baseline for EIR projects in a cost-optimal portfolio for a particular utility is determined, sensitivity analysis should be employed to test the impact of estimated Cargo Preference Act premiums.
Modeling guidance:
- Determine whether asset capex costs must be adjusted to reflect Davis-Bacon wages during construction. An adjustment may also be needed for maintenance capex where those are entered into the model.
- Regarding NEPA, solar and storage projects on previously disturbed land as well as transmission upgrades should, in the opinion of RMI, be treated as categorically excluded, resulting in negligible cost impacts and timing delays. For projects for which a categorical exclusion cannot be plausibly assumed, scenario analyses to account for EA and EIS cost and timing impacts would be reasonable; alternatively, these projects could be excluded from EIR financing treatment.
- For the Cargo Preference Act, RMI recommends modeling the EIR baseline without an explicit cost impact, followed by scenario analysis to test how potential incremental shipping costs might increase capex costs or affect assets and in turn reduce any PVRR advantage of an asset portfolio including EIR financing.
The EIR + Clean Repowering
Clean repowering savings potential will be highly site-specific. Modelers will need to estimate savings from reduced transmission interconnection costs while accounting for the associated operational constraints. A description of the four steps to modeling clean repowering in capacity expansion is available in the main text of this document; more detailed guidance is provided below.
Modeling guidance:
- Create site-specific opportunities for clean repowering resources at existing fossil fuel asset points of interconnection (POI). Wind, solar, and battery storage candidate resources should be made available at the POI, subject to physical and operational constraints.
- Physical constraints: RMI models the potential for unlimited battery storage connections and wind and solar connections capped at the capacities available within a given radius of existing fossil fuel assets, as per NREL’s solar and wind supply curve data. Other assumptions may be made to reflect on-the-ground project development realities known by the utility.
- Operational constraints: The combined output of existing thermal and new clean repowering resources should not exceed the maximum injection limit at the point of interconnection. Implementation of this constraint will vary across capacity expansion software. When possible, it should be done directly, by creating a joint constraint on hourly generation across the resources. It may also be possible to implement indirectly, by creating a new node (or equivalent) at the point of interconnection and instituting a transmission limit to the rest of the network with maximum flow equal to the point of interconnection injection limit. Note that the constraint should be placed on combined resource generation, not installed capacity, as the economically optimal solution to clean repowering may likely be to “overbuild” capacity behind the point of interconnection and use the hybrid resource flexibility to increase resource capacity factor. Enhanced availability from the hybrid resource is also likely to result in greater ability to meet peak demand, which should be reflected in the combined resource’s capacity accreditation.
- Reflect the value of clean repowering resources to mitigate interconnection delays. As described in the body of the main text, clean repowering resources are not subject to many of the interconnection delays faced by greenfield resources. In capacity expansion, the best way to model this is to remove any limits or constraints applied to annual capacity additions for clean repowering resources.
- Capture the economic benefits of clean repowering resources.
- One benefit of clean repowering resources is reduced transmission interconnection costs. In RMI’s modeling, these savings are captured by assuming the only transmission costs associated with the clean repowering resources are the costs of spur lines to connect to the existing thermal generation point of interconnection. Any other balance of system cost savings associated with developing hybrid rather than standalone resources should be reflected as well. All of these savings should be reflected in capacity expansion as reduced capital costs.
- Another economic benefit is the applicability of enhanced tax credits made available by the Inflation Reduction Act. In addition to the base value of the ITC or PTC, many clean repowering resources are likely to be eligible for the Energy Community Tax Credit Bonus given their proximity and intention connection to existing fossil infrastructure. This bonus credit provides up to an additional 10% of project costs if applied to the ITC or an additional 10% of the credit supplied by the PTC. Eligibility maps can be found on the program website. These savings should be modeled in capacity expansion as adjustments to resource capital costs.
- Finally, all clean repowering resources are eligible for EIR financing. These savings should be represented in capacity expansion the same as for other clean assets.
Endnotes
[1] E. Larson, C. Greig, J. Jenkins, E. Mayfield, A. Pascale, C. Zhang, J. Drossman, R. Williams, S. Pacala, R. Socolow, EJ Baik, R. Birdsey, R. Duke, R. Jones, B. Haley, E. Leslie, K. Paustian, and A. Swan, Net-Zero America: Potential Pathways, Infrastructure, and Impacts, Interim Report, Princeton University, 2020, https://netzeroamerica.princeton.edu/the-report.
[2] Support for this assumption is presented in Christian Fong, David Posner, and Uday Varadarajan, “Maximizing the Value of the Energy Infrastructure Reinvestment Program for Utility Customers,” RMI, May 24, 2024, https://rmi.org/maximizing-the-value-of-the-energy-infrastructure-reinvestment-program-for-utility-customers/.
[3] Docket No. E-100, Sub 190, “Testimony of Jeff Thomas Public Staff,” North Carolina Utilities Commission, May 29, 2024, https://starw1.ncuc.gov/NCUC/ViewFile.aspx?Id=eb84ecb4-3891-40a2-a7eb-426b153f1f3a.
[4] According to the US Department of Energy’s Title 17 Loan Program Guidance, to be eligible for EIR funding, investments need only “avoid, reduce, utilize, or sequester air pollutants and anthropogenic emissions of greenhouse gases,” which can include investments in generation resources, as well as a broad array of other technologies such as repowering or retooling energy infrastructure to operate more efficiently or at higher output. However, for the purposes of capacity expansion modeling, we recommend modelers at a minimum assume that all resources that do not emit greenhouse gases be defined as eligible for EIR funding within the model.
[5] Most clean resources selected by a capacity expansion model will likely qualify as “enabling existing energy infrastructure to reduce emissions,” but if these resources differ geographically from the location of existing infrastructure, more effort may be required to ensure compliance with EIR program eligibility requirements. The LPO website stipulates that EIR projects “must show a clear relationship between new services and benefits provided by the Title 17 financed infrastructure and services, and benefits lost from the legacy infrastructure that ceased operations, such as grid capacity, reliability, and workforce retention and opportunities, including if the replacement plan differs from the legacy infrastructure physically and/or geographically.” See https://www.energy.gov/lpo/energy-infrastructure-reinvestment.
[6] Docket U-240013, “Policy Statement Addressing the Federal Inflation Reduction Act and the Infrastructure Investment and Jobs Act in Utility Planning,” Washington Utilities and Transportation Commission, May 3, 2024, https://apiproxy.utc.wa.gov/cases/GetDocument?docID=31&year=2024&docketNumber=240013.
[7] Clean Repowering: How to Capitalize on Fossil Grid Connections to Unlock Clean Energy Growth, RMI, 2024, https://rmi.org/insight/clean-repowering/.
[8] Katie Siegner and Alex Engel, “Clean Repowering: A Near-Term, IRA-Powered Energy Transition Accelerant,” RMI January 16, 2024, https://rmi.org/clean-repowering-a-near-term-ira-powered-energy-transition-accelerant/.
[9] The cost and benefit calculation for project delays is a complex discounting equation. All else equal, delaying deployment should reduce the present value of costs due to discounting. However, there can be cost increases associated with construction financing loan extension, or other payments required for project delays. Additionally, we assume cost of deploying clean energy will decrease as time progresses, but it is uncertain whether the cost savings of postponed deployment will exceed the known savings associated with the EIR program, under which funds are available to be committed between now and September 2026 for projects (or project milestones) that must be completed by September 31, 2031.
[10] “Grid connection backlog grows by 30% in 2023, dominated by requests for solar, wind, and energy storage,” Berkely Lab, April 10, 2024, https://emp.lbl.gov/news/grid-connection-backlog-grows-30-2023-dominated-requests-solar-wind-and-energy-storage.
[11] The Synapse report “No-Regrets Solutions for Accelerating Grid Interconnection How Fast-Track Interconnection Processes Cut Costs in SPP and MISO” provides a real world example of the interconnection cost savings that can be achieved through clean repowering. A solar project in Sumner County, Kansas, had total interconnection costs before construction of approximately $333/kW, of which roughly 70% were for transmission network upgrades, with a wait of more than eight years to interconnect. In contrast, a solar project in Harper County, Kansas, used surplus interconnection, and there were no transmission network upgrades associated with this project, resulting in less than $1/kW total interconnection costs before construction.
[12] RMI’s article “For Ratepayers to Realize Savings from Clean Energy, Utility Business Models Need an Update” elaborates on the ways in which taking advantage of IRA opportunities is misaligned with traditional utility incentives.
[13] As described in documents from the 2021 NARUC-NASEO Comprehensive Electricity Planning Framework Taskforce and 2021 FERC Joint Federal-State Task Force on Electric Transmission. For further discussion and examples of leading practices, see Mark Dyson, Lauren Shwisberg, and Katerina Stephan, Reimagining Resource Planning, RMI, January 2023, https://rmi.org/insight/reimagining-resource-planning/.
[14] Christian Fong, David Posner, and Uday Varadarajan, “The Energy Infrastructure Reinvestment Program: Federal financing for an equitable, clean economy,” RMI, February 16, 2024, https://rmi.org/the-energy-infrastructure-reinvestment-program-federal-financing-for-an-equitable-clean-economy/.
[15] The 2014 GAO report also noted that 95% of DOE’s 9,060 NEPA analyses from 2008 to 2012 were categorical exclusions; ibid., 7.
[16] DOE NEPA Categorical Exclusion Rulemaking, DOE, 2024, https://www.energy.gov/sites/default/files/2024-04/doe-10-cfr-1021-final-rule-2024-04-30-final.pdf.
[17] National Environmental Policy Act: Little Information Exists on NEPA Analyses, GAO-14-369, April 15, 2014, https://www.gao.gov/products/gao-14-370.
[18] LPO’s Energy Infrastructure Reinvestment (1706) Program https://www.energy.gov/lpo/articles/lpos-energy-infrastructure-reinvestment-1706-program
[1] E. Larson, C. Greig, J. Jenkins, E. Mayfield, A. Pascale, C. Zhang, J. Drossman, R. Williams, S. Pacala, R. Socolow, EJ Baik, R. Birdsey, R. Duke, R. Jones, B. Haley, E. Leslie, K. Paustian, and A. Swan, Net-Zero America: Potential Pathways, Infrastructure, and Impacts, Interim Report, Princeton University, 2020, https://netzeroamerica.princeton.edu/the-report.
[2] Support for this assumption is presented in Christian Fong, David Posner, and Uday Varadarajan, “Maximizing the Value of the Energy Infrastructure Reinvestment Program for Utility Customers,” RMI, May 24, 2024, https://rmi.org/maximizing-the-value-of-the-energy-infrastructure-reinvestment-program-for-utility-customers/.
[3] Docket No. E-100, Sub 190, “Testimony of Jeff Thomas Public Staff,” North Carolina Utilities Commission, May 29, 2024, https://starw1.ncuc.gov/NCUC/ViewFile.aspx?Id=eb84ecb4-3891-40a2-a7eb-426b153f1f3a.
[4] According to the US Department of Energy’s Title 17 Loan Program Guidance, to be eligible for EIR funding, investments need only “avoid, reduce, utilize, or sequester air pollutants and anthropogenic emissions of greenhouse gases,” which can include investments in generation resources, as well as a broad array of other technologies such as repowering or retooling energy infrastructure to operate more efficiently or at higher output. However, for the purposes of capacity expansion modeling, we recommend modelers at a minimum assume that all resources that do not emit greenhouse gases be defined as eligible for EIR funding within the model.
[5] Most clean resources selected by a capacity expansion model will likely qualify as “enabling existing energy infrastructure to reduce emissions,” but if these resources differ geographically from the location of existing infrastructure, more effort may be required to ensure compliance with EIR program eligibility requirements. The LPO website stipulates that EIR projects “must show a clear relationship between new services and benefits provided by the Title 17 financed infrastructure and services, and benefits lost from the legacy infrastructure that ceased operations, such as grid capacity, reliability, and workforce retention and opportunities, including if the replacement plan differs from the legacy infrastructure physically and/or geographically.” See https://www.energy.gov/lpo/energy-infrastructure-reinvestment.
[6] Docket U-240013, “Policy Statement Addressing the Federal Inflation Reduction Act and the Infrastructure Investment and Jobs Act in Utility Planning,” Washington Utilities and Transportation Commission, May 3, 2024, https://apiproxy.utc.wa.gov/cases/GetDocument?docID=31&year=2024&docketNumber=240013.
[7] Clean Repowering: How to Capitalize on Fossil Grid Connections to Unlock Clean Energy Growth, RMI, 2024, https://rmi.org/insight/clean-repowering/.
[8] Katie Siegner and Alex Engel, “Clean Repowering: A Near-Term, IRA-Powered Energy Transition Accelerant,” RMI January 16, 2024, https://rmi.org/clean-repowering-a-near-term-ira-powered-energy-transition-accelerant/.
[9] The cost and benefit calculation for project delays is a complex discounting equation. All else equal, delaying deployment should reduce the present value of costs due to discounting. However, there can be cost increases associated with construction financing loan extension, or other payments required for project delays. Additionally, we assume cost of deploying clean energy will decrease as time progresses, but it is uncertain whether the cost savings of postponed deployment will exceed the known savings associated with the EIR program, under which funds are available to be committed between now and September 2026 for projects (or project milestones) that must be completed by September 31, 2031.
[10] “Grid connection backlog grows by 30% in 2023, dominated by requests for solar, wind, and energy storage,” Berkely Lab, April 10, 2024, https://emp.lbl.gov/news/grid-connection-backlog-grows-30-2023-dominated-requests-solar-wind-and-energy-storage.
[11] The Synapse report “No-Regrets Solutions for Accelerating Grid Interconnection How Fast-Track Interconnection Processes Cut Costs in SPP and MISO” provides a real world example of the interconnection cost savings that can be achieved through clean repowering. A solar project in Sumner County, Kansas, had total interconnection costs before construction of approximately $333/kW, of which roughly 70% were for transmission network upgrades, with a wait of more than eight years to interconnect. In contrast, a solar project in Harper County, Kansas, used surplus interconnection, and there were no transmission network upgrades associated with this project, resulting in less than $1/kW total interconnection costs before construction.
[12] RMI’s article “For Ratepayers to Realize Savings from Clean Energy, Utility Business Models Need an Update” elaborates on the ways in which taking advantage of IRA opportunities is misaligned with traditional utility incentives.
[13] As described in documents from the 2021 NARUC-NASEO Comprehensive Electricity Planning Framework Taskforce and 2021 FERC Joint Federal-State Task Force on Electric Transmission. For further discussion and examples of leading practices, see Mark Dyson, Lauren Shwisberg, and Katerina Stephan, Reimagining Resource Planning, RMI, January 2023, https://rmi.org/insight/reimagining-resource-planning/.
[14] Christian Fong, David Posner, and Uday Varadarajan, “The Energy Infrastructure Reinvestment Program: Federal financing for an equitable, clean economy,” RMI, February 16, 2024, https://rmi.org/the-energy-infrastructure-reinvestment-program-federal-financing-for-an-equitable-clean-economy/.
[15] The 2014 GAO report also noted that 95% of DOE’s 9,060 NEPA analyses from 2008 to 2012 were categorical exclusions; ibid., 7.
[16] DOE NEPA Categorical Exclusion Rulemaking, DOE, 2024, https://www.energy.gov/sites/default/files/2024-04/doe-10-cfr-1021-final-rule-2024-04-30-final.pdf.
[17] National Environmental Policy Act: Little Information Exists on NEPA Analyses, GAO-14-369, April 15, 2014, https://www.gao.gov/products/gao-14-370.
[18] LPO’s Energy Infrastructure Reinvestment (1706) Program https://www.energy.gov/lpo/articles/lpos-energy-infrastructure-reinvestment-1706-program