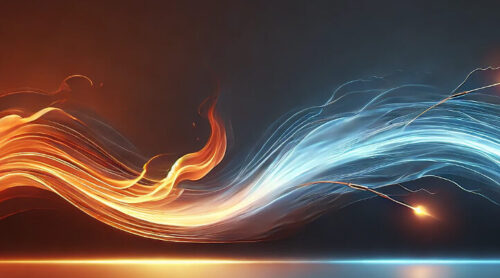
Energy after Fire
The shift from primitive heat to modern work
Introduction
Our energy system is stuck in the past. Fire has been our primary source of energy for over a million years, providing the essential heat needed to survive. This reliance on fire made sense when our principal energy needs were purely for heat. However, today’s energy demands have evolved far beyond this primal necessity. Unlike in past millennia, we now require more work than heat: we desire mobility, motors, electrical appliances, and data processing in greater quantities than we do warmth. Despite this transformation over the past century from heat demand to work demand, our fundamental energy supply methods have not changed much, and are still mostly heat generation. This has led to incredible inefficiency, which we describe in a prior article.
We need energy sources fit for an era of work demand, not heat demand. Fortunately, thanks to the rapid growth and cost decline of solar, wind, and electrification, “firepower” faces inexorable decline.
Summary
- New energy for a new era. As we transition from fossil fuels to renewables and electric cleantech, our energy system is set to nearly double in primary-to-useful energy efficiency. This is driven by the fundamental physics of heat and work.
- A simple way to look at the complex energy system. Energy supply comes as either heat (from burning fuels like coal and gas) or work (from moving electrons with hydro, solar, and wind). The energy services we require mirror this: some require heat (e.g., industry and building heat), others work (e.g., transport and engines). Heat sources are good at providing heat (50%–70+% efficient), and work sources excel at delivering work (70+% efficient).
- The heat and work mismatch. Today, over 95% of our energy comes from heat supply, yet most of what we need is work. But converting heat to work is only about 33% efficient on average, and given the laws of thermodynamics, it cannot get much higher. This leads to massive energy losses of over 200 EJ per year, making up nearly 60% of all global energy waste.
- A century of growing supply-demand divergence. A hundred years ago, our energy needs were mostly for heat, so sourcing mostly heat supply made sense. While our needs shifted to mostly work services today, our energy supply remained mostly heat, leaving us with a century’s worth of compounded inefficiency.
- We found a solution: renewables get straight to work. Renewables generate electricity directly, bypassing the inefficient conversion from heat to work. This allows them to outperform traditional energy sources while boosting overall efficiency.
- A leap in energy productivity is coming. As renewables replace fossil fuels, we’ll see a massive, once-in-a-century leap in energy productivity — similar to the post-World War II boom when oil and gas took over from coal and biomass.
Heat and work
Today’s energy system is vastly complex. We use dozens of different fuels to deliver hundreds of different energy services — to heat water, to move cars, to produce steel, and to process data. Inspired by the work of Nick Eyre, we can take a bird’s eye view that reveals a simple dichotomy: both the energy services we demand and the fuels we put into the energy system can be split into two groups: heat and work.[1]
Let’s first look at energy services. Fundamentally, all energy demand comes down to either heating things up or making things move (in physics terms, doing “work”). Consider taking a hot shower or driving a car: the former requires heating water, and the latter requires setting wheels in motion. Even for services where it is less obvious, such as lighting a room or running a data center, the same principle applies. An incandescent lightbulb illuminates by heating up a filament until it glows, while running data centers involves sending targeted pulses of electricity through a hard drive or a microchip to move bits from 0 to 1. Hereinafter, we refer to this distinction as heat or work services.
On the supply side, we see a similar split. All energy sources release their energy in one of two ways: by heating up their surroundings, or by making something else move (in physics terms, “doing work” by “exerting a force”). The former is most common — coal, oil, and gas all consist of molecules where energy is stored in molecular bonds that can be broken by reacting with oxygen, resulting in the release of heat through combustion. This method of supplying energy — setting things on fire to generate heat — we call heat supply. Fire has been the mainstay of our energy system for centuries.[2]
However, there is another type of energy source that releases its energy by making things move rather than releasing heat. For example, renewables such as wind and solar convert wind gusts and sunrays into energized, moving electrons — in physics terms, they exert a force through the electromagnetic field. This force can travel along a power cable and make other electromagnetically active materials move; for instance, a magnetic rotor in an engine. In this case, energy is released not as heat but as directed motion. We define solar PV, wind and hydro as sources of work supply.
So at a high level, energy supply simplifies to energy sources that release heat — typically through combustion, or energy sources that do targeted work — typically through electromagnetic forces. As Amory Lovins aptly puts it, “fiery molecules or obedient electrons.”[3]
Unsurprisingly, heat energy supply tends to be relatively good at providing heat services, and work energy supply is good at doing work. Today, the average efficiency of turning primary heat fuel into useful heat is about 50% globally, but can be upward of 70% for more efficient heating equipment. The efficiency of turning work supply into work services is about 70% on average. In contrast, turning heat supply into work services is incredibly inefficient. For instance, burning oil to power a car or combusting coal to make electricity to run a pump is only just over 30% efficient on average.
Exhibit 1: Global energy flow from supply to demand split by heat and work
A century of growing supply-demand mismatch
Just over a century ago, the vast majority of our energy service needs were for heating, making up almost 90% of useful energy demand. Fiery molecules were a fine match, and effectively all energy supply came from heat fuels such as biomass and coal.
As humanity got better at harnessing heat supply, and found cheaper and denser forms, it unlocked new energy services that were previously unimaginable, such as personal cars and air travel. The ability to convert heat into work spread across the world and drove a significant shift in demand. As people sought more advanced energy services — more transportation, automation, and electronics — the demand for work services skyrocketed.
However, despite the evolution of the share of energy service needs from heat to work, our fundamental energy supply mix remained stagnant. Technologies such as combustion engines and power plants enabled us to provide new work services, but they did so through the inherently inefficient process of converting heat fuels into work. To keep up with rising work service demands, we dug ever deeper for more heat supply, fueling an era of unprecedented growth in prosperity, but at the expense of incredible energy waste.
Today, even though the majority of our energy demand is for work, over 95% of our energy supply still comes from heat sources. We supplied a boom in new energy services, but did so inefficiently with the heat fuels we had on hand, leading to an ever-increasing buildup of energy waste, as shown in Exhibit 3.
The mismatch in energy supply and demand is, of course, in part driven by the fact that alternative work sources were not yet readily available. For example, the first solar panel was made only in the 1950s at Bell Labs, became economical for mass markets just a decade ago, and we are still finding new ways to integrate solar today. So over the past century, there weren’t many ways for the world to move from heat to work supply, nor did incumbent energy suppliers look very hard. As a result, inefficiencies compounded.
Exhibit 2: A hundred years of heat energy supply and demand
Whether by necessity or intention, we built up a profound mismatch between the type of energy supplied (heat) and the type of energy service desired (work). We made things work, but only by accepting significant inefficiency. We are well overdue for a tech update to fix century-old issues that have been building up in our energy system—because, as shown below, over 60% of all energy losses today come from technologies clumsily trying to convert heat supply into work demand. Wrong tool for the job.
As the demand for work services grew, so our energy waste rose. As we note in a previous article, two technologies make up a particularly large share of energy waste: internal combustion engines for transport and thermal power plants to drive electric equipment make up some 85% of all heat-to-work energy waste.
Exhibit 3: A hundred years of energy waste
Our last major update was in the 1970s
Looking at the incredible inefficiency of today’s energy system, it may be hard to believe we’ve actually become more efficient than a century ago. Over time, we’ve become better at turning heat supply into energy services. Where the primary-to-useful efficiency (the ratio of energy output to energy input) of the global energy system in 1900 was only about 20%, it is about 35% today.
This evolution did not happen gradually but occurred over a short, three-decade burst post-World War II, as shown below. The driver of this change was a major update to the energy system, transitioning from biomass and coal in primitive energy technologies, such as fireplaces and locomotives, to gas and oil in more advanced technologies, such as gas boilers and internal combustion engines. The result was a boom in energy productivity.
However, once this technology transformation was complete, primary-to-useful efficiency gains stagnated again. Economic energy intensity — measured as primary energy consumption relative to GDP — continued to improve, but mainly due to advancements in end-use efficiency. The primary-to-useful energy efficiency has remained flat at around 35% since the 1970s. This means that nearly two-thirds of the total energy fed into the system produces no value. While new technologies have become marginally more efficient, no major breakthroughs have been achieved as we near the theoretical limits of converting heat into work. That’s because no matter how hard one tries, it is thermodynamically impossible to get much more than a third to half of a heat fuel converted into work. We’ve gone as far as fire can take us.
Exhibit 4: Global energy system efficiency since 1900
Renewables get straight to work
Burning gas to light a room creates more heat than light. Burning coal to create electricity creates more heat than electricity. Burning oil to move a vehicle creates more heat than motion. We are sending more energy up smokestacks and out exhaust pipes than we are putting to work to power our economy.
This is where a new generation of energy sources comes in. Wind and solar directly generate electricity without heat-to-work conversion losses, and hence produce energy that can get straight to work. Electric motors embedded in a new generation of clean technologies, such as EVs, can turn renewable electrons into motion and work at well over 90% efficiency. This makes it dramatically easier to supply work services since we start with work supply to begin with. It means we can get work done without the incredible losses of first converting heat to work.
Renewables and electrification are the basic updates to the energy system we sorely need.
Work beats heat even for heat services
Solar and wind renewables are clearly well positioned to scale into a large market: as work energy supply, they can easily replace wasteful heat supply to meet society’s work services, which is over half of total energy needs today and rising.
But it does not stop there. Perhaps surprisingly, getting heat services out of work supply is much more efficient than the other way around. The reason for this lies at the atomic level: both heat and work are just atomic motion; heat is random motion, while work is directional, organized motion, with all atoms moving in unison. Seen through this lens, it becomes clear why turning heat into work is harder than work into heat: anyone who has seen a school class line up before and after recess realizes that turning orderly motion into chaotic, undirected motion is much easier than turning chaotic motion back into order — as with kids, so for atoms. It is always easier to start with order: turning work supply into heat services tends to be more efficient than turning heat supply into heat services. For example, renewable-powered heat pumps are about three times more efficient than gas boilers at heating homes.
Thus, now that we have cheap sources of work supply readily available, such as wind and solar power, it stands to reason that we won’t just see all work services starting to use it, but a large share of heat services as well. Of the total 48% of energy demand for heat services today, over half is for low-temperature (<120°C) heating, which is well suited for efficient heat pumps. All of these heat services can move to work supply with tremendous efficiency gains. Even higher-temperature heat services can be in play: electric furnaces can easily reach, if not exceed, the same efficiency as gas furnaces.
This means that the cleantech revolution won’t just push fossil fuels out of work services, but can also challenge and replace them across different heating services.
Another quantum leap in energy productivity is coming
As renewables and cleantech surge, we can expect to see another quantum leap in global energy productivity. As shown in Rystad’s energy scenarios below, another step jump in efficiency is coming, much like post-World War II. As our previous article explains, three drivers of change will make the difference: electrification, localization, and renewables.
Note that all of these efficiency gains are before even considering end-use efficiency. In this article, we’ve only looked at the ratio of useful energy for services over primary supply. Of course, we can also get much smarter in how we turn energy services into prosperity and satisfaction— think for instance about better logistics that help trucks to avoid driving excess miles, or smart heating that avoids heating empty homes.
Exhibit 5: Past, present and future global energy system efficiency
As efficient electrons take over work services as well as a rising share of heat services, work supply becomes the dominant energy provider, leading to a once-in-a-century boom in energy productivity. This means each unit of primary energy will go further: lighting more darkness, energizing more lives, and generating greater prosperity. And as we decouple growth from inefficient heat supply, productivity will boom, and energy waste will, for the first time in a century, begin to decline.
Exhibit 6: Energy waste and economic growth past and future
Conclusion
For over a century, we’ve been using the hammers of heat supply to bash in the screws of work demand. We got quite good at it and made it work — as long as you ignored the incredible inefficiency of the botched job. Now, with renewable electricity, we’ve found a screwdriver. As we match the tool to the task, we can rapidly become more productive and power society with energy sources that actually work for us. And that means we are the dawn of a historic transition where humanity parts ways with fire as its main energy source.
A few decades from now, we will look back at using so much heat supply and view it as an oddity. It will seem as outdated as using gas to light our streets, or open smoky fires to warm homes — once normal, now simply outdated.
While the shift from heat supply to work supply is inevitable, the timing is not. The question now is whether we can retool our energy reliance from heat to work fast enough to prevent the emissions of burning stuff from frying the planet.
Endnotes
RMI acknowledges the support from the Energy Demand changes Induced by Technological and Social innovations (EDITS) project, which is part of the initiative coordinated by the Research Institute of Innovative Technology for the Earth (RITE) and International Institute for Applied Systems Analysis (IIASA) (and funded by Ministry of Economy, Trade, and Industry (METI), Japan).
[1] A similar principle applies to nuclear energy, although instead of breaking molecular bonds, we break atomic bonds in the cores of heavy elements using neutrons rather than oxygen. Nonetheless, the result is the same: energy is released, ultimately as heat.
[2] See N. Eyre (2021) “From using heat to using work: reconceptualising the zero carbon energy transition,” available at https://link.springer.com/article/10.1007/s12053-021-09982-9, or Hannah Ritchie’s great summary of Nick Eyre’s work at https://www.sustainabilitybynumbers.com/p/electrification-energy-efficiency.
[3] For a more detailed overview of the implications of this, see A. Lovins (2016), “Reinventing Fire.”